Whence Came These Plants Most Foul? Phylogenomics and Biogeography of Lowiaceae (Zingiberales)
- 1Singapore Botanic Gardens, National Parks Board Singapore, Singapore, Singapore
- 2Department of Biological Sciences, International Center for Tropical Botany, Institute of Environment, Florida International University, Miami, FL, United States
- 3National Tropical Botanical Garden, Kalāheo, HI, United States
- 4School of Biological Sciences, Nanyang Technological University, Singapore, Singapore
- 5Department of Botany, National Museum, Prague, Czechia
- 6Royal Botanic Garden Edinburgh, Edinburgh, United Kingdom
Lowiaceae (order Zingiberales) is a small family of forest herbs in Southeast Asia. All species belong to the genus Orchidantha. They are known for possessing orchid-like flowers that are smelly, apparently mimicking dead animals, feces, or mushrooms. Little is known of the biogeographic patterns or character evolution of the family. We sampled the family extensively, including many recently discovered species, and reconstructed the phylogeny of the family using HybSeq with Lowiaceae-specific RNA baits. Our phylogenetic reconstructions confirm that the family is most closely related to Strelitziaceae, and that species with dark, foul-smelling flowers form a grade in which a clade of species with paler flowers are embedded. The pale-flowered species produce a distinct odor, resembling edible mushrooms. Apart from a single species, the species from Borneo form a clade, and the same is true for Indochinese species. The remaining species form a more widespread clade. A biogeographic analysis shows that the distribution of Lowiaceae can explained by vicariance and gradual dispersal from a shared ancestral range of Borneo and Indochina. There is no evidence of long-distance dispersal, only a later extension in distribution to Peninsular Malaysia which coincides with the presence of a land bridge. Different directions of spread are possible, but none require long-distance dispersal. The results are consistent with the geological history of Southeast Asia. In particular, the relatively early isolation between Indochina and Borneo could be explained by the presence of a sea barrier that developed 10–15 MYA, and the continuous movement of plant species between Borneo and Peninsular Malaysia could be explained by a land bridge that existed until c. 5 MYA. The lack of an extensive land bridge with a suitable habitat may explain the absence of this genus from Sumatra and other Indonesian islands aside from Borneo. The strict reliance on a continuous habitat for the range expansion of Lowiaceae can be explained by their fruits and seeds, which lack obvious adaptations for long-distance dispersal. The inability to disperse to new areas may also explain why the extant species have very restricted distributions.
Introduction
Lowiaceae Ridl. (Zingiberales), with a single genus Orchidantha N. E. Brown (1886), is a small family of forest herbs. The family belongs to the so-called “banana group” of Zingiberales, comprising families that have five or six fertile stamens (Musaceae, Heliconiaceae, Strelitziaceae and Lowiaceae). The remaining families of the order belong to the “ginger group,” characterized by a single fertile or partially fertile stamen. The relationship of the families in Zingiberales were reviewed and studied by Carlsen et al. (2018). They report that genetic analyses have consistently placed Lowiaceae as sister to the Gondwanan family Strelitziaceae (Kress et al., 2001; Givnish et al., 2006; Barrett et al., 2014; Sass et al., 2016; Carlsen et al., 2018; the same relationship was recovered by Smith et al., 1993; Liang et al., 2020, not reviewed by Carlsen et al., 2018); they report only one study reporting a conflicting topology (Kress, 1990) made using a morphological character matrix. Johansen (2005) found that Lowiaceae is sister to the rest of Zingiberales, but with low support. Kirchoff et al. (2020) found similarities in the inflorescence structure and flower development of Lowiaceae and Strelitziaceae that support their sister relationship. In distribution, Lowiaceae is restricted to the Malesian and Indochinese floristic regions sensu Takhtajan (1986) and as refined by Welk (2015), specifically from southernmost China to Borneo. In most phylogenetic studies, Lowiaceae has been poorly represented, often by a single sample. The only previous study on the molecular phylogeny and phylogeography within Lowiaceae was based on 14 species (including two then-undescribed taxa) and six markers (Johansen, 2005). Johansen concluded that the species from Borneo (strongly supported to be monophyletic), are sister to the rest of the genus (sister relationship poorly supported). Although the species coverage was extensive for Borneo and the Thai-Malay Peninsula, only a single species that occurs above the Isthmus of Kra, O. chinensis T.L.Wu, was included. At that time, the two species from Indochina with the most obvious morphological similarity to the Bornean species were known only from historical collections. Based on sea-level changes over time, Johansen (2005) suggested that Lowiaceae has an origin in the northeastern parts of Sundaland, from where it dispersed to Indochina, the Thai-Malay Peninsula and Borneo.
Lowiaceae are mostly restricted to shady and moist locations in lowland primary forests. The family was last revised by Holttum (1970), who recognized six species. Since then about 27 species have been described, mostly from Borneo (e.g., Larsen, 1993; Nagamasu and Sakai, 1999; Pedersen, 2001; Syauqina et al., 2016, 2019; Poulsen and Leong-Škorničková, 2017; Leong-Škorničková et al., 2021), but also from Peninsular Malaysia (Leong-Škorničková, 2014), Thailand (Jenjittikul and Larsen, 2003), Vietnam (Trần and Leong-Škorničková, 2010; Leong-Škorničková et al., 2014; Trần et al., 2020) and China (Fang and Qin, 1996; Zou et al., 2017, 2019). Lowiaceae collections are sparse in herbaria, and are likely severely under-collected as the plants are rare and often sterile. The zygomorphic flowers appear at ground level where they are well camouflaged among the leaf litter and are hard to spot. It is therefore possible that many more species are yet to be discovered.
Vegetative morphology is rather uniform across the genus, in most instances making species-level identification impossible in the absence of flowers. Based on leaf morphology, the species can be divided into two groups: Species with petiolate leaves vs. those with the lamina tapering gradually down to the leaf sheath. Young individuals of most (if not all) species have petiolate leaves, so it is necessary to ensure that only fully mature individuals are assessed for this character.
Due to the lack of useful vegetative characters, flower morphology is critical for species recognition. Two main flower types can be recognized based on sepal position. In the “claw” type, the lateral sepals support the labellum, often partially overlapping or even crossing each other, while the dorsal sepal arches over the labellum, giving the flower the appearance of a crab claw (see Figures 1A–E,G,I, 2A–D,F). In the second type, here called the “propeller” type, the lateral sepals spread without supporting the labellum, and the three sepals together form a shape resembling a three-blade propeller (see Figures 1F–H, 2E, 3A–F). Additionally, flowers can be divided into three categories based on the color of the labellum. The majority of species belonging to the “dark” type have a very dark purple to purple labellum, occasionally mixed with yellow, pale purple or cream (Figures 1A–F,H,I, 2), with the sole exception of O. lutescens Škorničk. and A.Lamb, which has a pale yellow labellum with purple shading at its base (Figure 1G). The “white” type of flower usually has a predominantly pure white or cream white (Figures 3A,D–F) labellum, while the “puce” type has a light puce to a rusty brown labellum (Figures 3B,C).
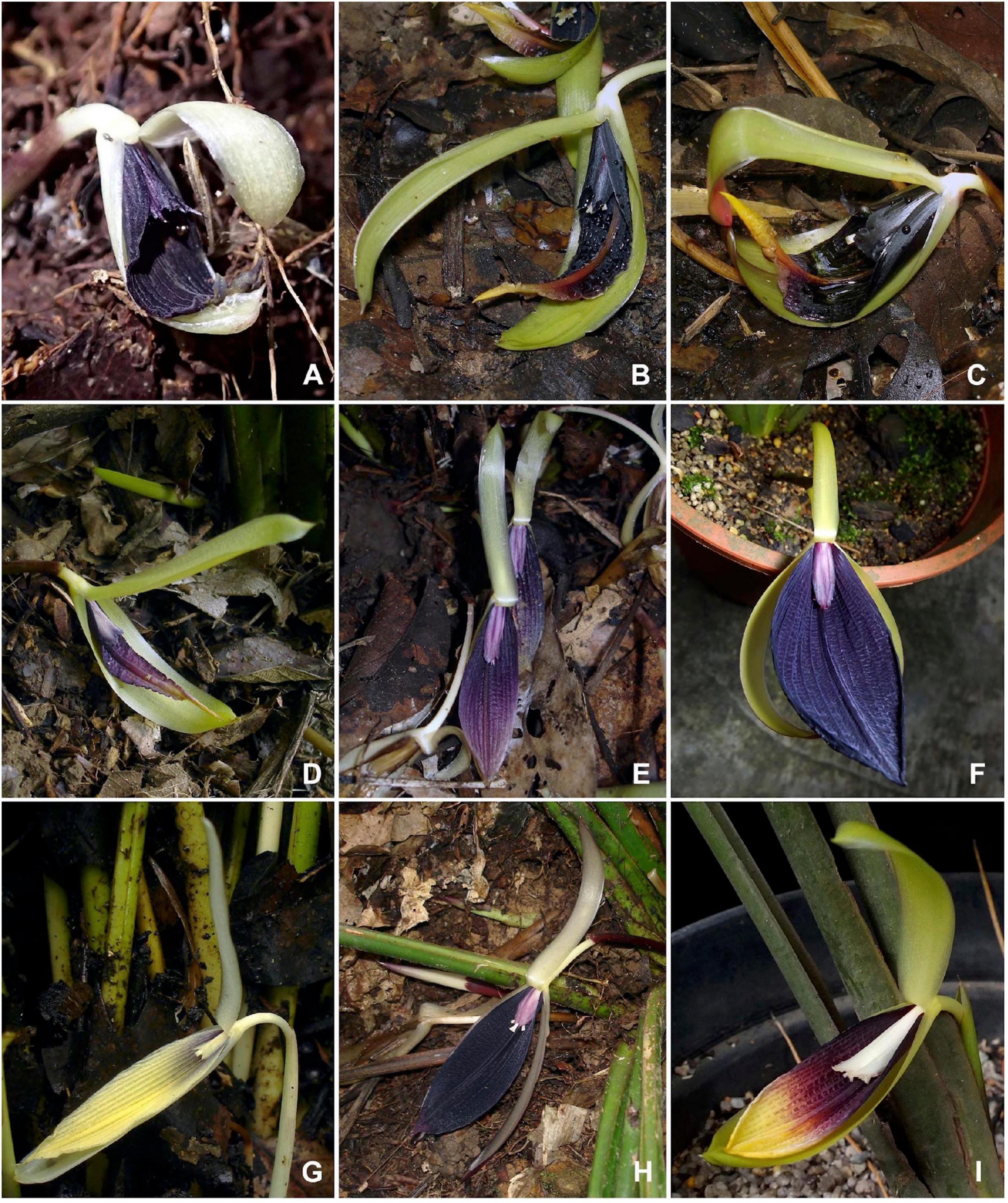
Figure 1. Diversity of Orchidantha in Borneo. (A) O. micrantha. (B) O. suratii. (C) O. holttumii. (D) O. sabahensis. (E) O. ultramafica. (F) O. grandiflora. (G) O. lutescens. (H) O. nilusii. (I) O. quadricolor.—Photo credits: (A) Axel Dalberg Poulsen. (B–I) Jana Leong-Škorničková.
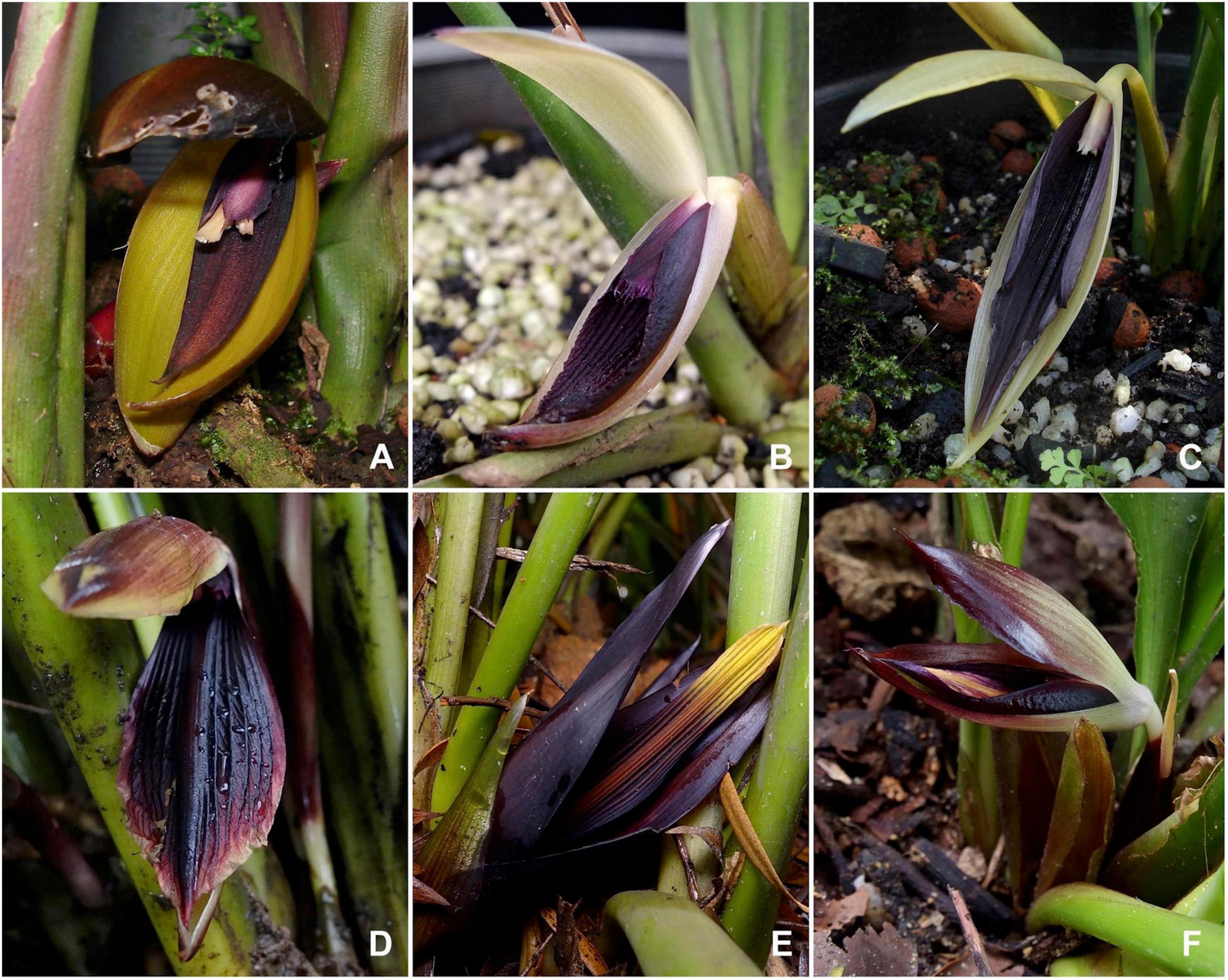
Figure 2. Diversity of Orchidantha in the Indochinese region. (A) O. foetida. (B) O. stercorea. (C) O. vietnamica. (D) O. virosa. (E) O. chinensis. (F) O. laotica.—Photo credits: (A–F) Jana Leong-Škorničková.
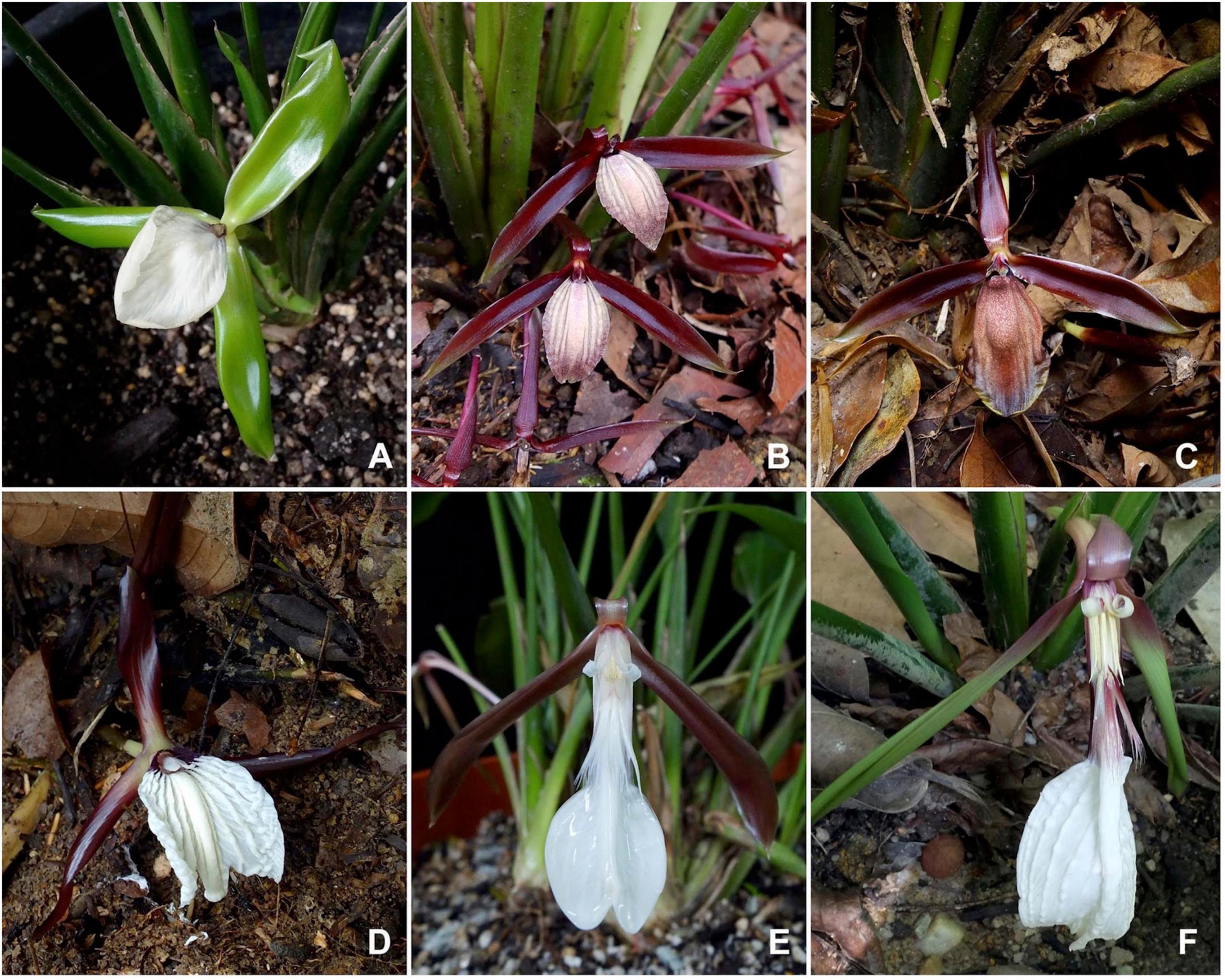
Figure 3. Diversity of Orchidantha with white and puce labella (Peninsular Malaysia and southernmost Thailand, with the exception of O. jiewhoei which is from Borneo) (A) O. jiewhoei. (B) O. maxillarioides. (C) O. lengguanii. (D) O. calcarea. (E) O. siamensis. (F) O. fimbriata. Photo credit: (A–F) Jana Leong-Škorničková.
Lowiaceae are known for their complicated stigma morphology (Pedersen and Johansen, 2004) which plays a major role in a sophisticated deceit pollination system involving beetles. Most species emit what can only be described as a truly awful smell, often mimicking a dead animal or excrement. Pollination studies on Lowiaceae are scarce and have largely been restricted to species with dark labella, but these have unanimously confirmed pollination by dung, carrion, or scavenger beetles attracted by the odor of dung or rotten meat, a syndrome known as coprocantharophily (Knoll, 1926; Faegri and van der Pijl, 1971; Beath, 1996, 1999; Sakai and Inoue, 1999; Gibernau et al., 2004; Grimm, 2009). Diurnal pollination by scarabaeid dung beetles from the genus Onthophagus Latreille was first discovered and described in detail from Borneo on Orchidantha inouei Nagam. and Sakai and Inoue (1999). Three additional Bornean species, Orchidantha grandiflora Mood and L.B.Pedersen, O. quadricolor L.B.Pedersen and O. suratii L.B.Pedersen were also reported to be pollinated by three different species of Onthophagus beetles, suggesting high species-specific dependence between plant and pollinator (Pedersen, 2003). More recently, Vislobokov et al. (2017) reported nocturnal pollination by carrion beetles from the genus Phaeochrous Castelnau in O. virosa Škorničk. and Q.B.Nguyen in Vietnam. The pollination of species with white- and puce-colored labella was studied in Peninsular Malaysia by Pedersen (2003), who concluded that fruit beetles from the genera Triacanus Erichson and Pocadius Erichson, both from Nitidulidae, are involved in the pollination of O. fimbriata Holttum and O. maxillarioides (Ridl.) K.Schum. respectively (Pedersen, 2003). Nitidulidae are also involved in pollination of O. jiewhoei Škorničk. and A.Lamb (Leong-Škorničková et al., 2021; see Figures 6E,F therein), the only white-flowered species in Borneo. Beetles from Nitidulidae are often associated with fruity and yeasty/fermentation floral odors (Goodrich and Raguso, 2009) as well as puffball mushrooms, and indeed the labella in the white- and puce-labella species often smell like mushrooms (usually partly decomposed mushrooms) supplemented by various smells of mildly rancid coconut oil and sweat.
Chromosome counts are known for seven species so far. Of these, five are presumed to be diploids with 2n = 18 (x = 9): Orchidantha maxillarioides (Larsen, 1966; Mahanty, 1970; Song et al., 2003, 2004), O. longiflora (Scort.) Ridl (Mahanty, 1970), O. siamensis K. Larsen (C. Artharamas pers. comm. in Larsen, 1996), O. holttumii K. Larsen (Larsen, 1993) and O. insularis T.L.Wu (Song et al., 2003, 2004). Song et al. (2003, 2004) reported counts of O. chinensis and O. longisepala D.Fang as 2n = 54, implying hexaploidy.
The genome size of Lowiaceae is known only for a single species, O. maxillarioides. It was first reported by Bharathan et al. (1994) as 2C = 7.10 pg (PI flow cytometry, chicken red blood cells and Nicotiana tabacum L. as standard), and more recently by Šmarda et al. (2014) as 2C = 6.86 pg (PI flow cytometry, Pisum sativum L. as standard).
Aims
In the present study, we produce the most taxonomically, geographically and genetically comprehensive phylogenetic reconstruction of Lowiaceae to date. Since the work of Johansen (2005), the number of described species in Lowiaceae has doubled. We include many of the recently described species, and improve sampling from Indochina. We use HybSeq to analyze thousands of markers across the nuclear genome. We use dated phylogenies to reconstruct the biogeographical history of the genus, and use the reconstructed phylogenetic relationships to estimate the number of dispersal events in the family. We also map morphological and genomic characters (chromosome numbers and genome size) to evolutionary lineages in order to find possible morphological and genomic synapomorphies as well as ancestral character states. Based on these analyses, we assess the possibility of subgeneric classification, particularly in relation to the main flower types in the genus. We also investigate the evolution and diversity of genome sizes and ploidy levels, and discuss how species discovery affects phylogeographic analyses.
Materials and Methods
Plant Material
Material for DNA extraction was obtained from tissue collected in the field and from the living and herbarium collections of Singapore Botanic Gardens (Supporting Information—Supplementary Table 1). A total of 48 samples, which included 42 samples of Lowiaceae (representing 27 accepted species and one unpublished taxon) and 6 outgroup species, were sampled for this study (Supplementary Table 1). The main dataset was used for the initial phylogenetic reconstruction, after which we selected one sample per taxon for downstream analyses (28 Lowiaceae samples and 6 outgroup samples). Approximately 80% of the currently known diversity of the genus was sampled, and the selection of Lowiaceae species was targeted to cover the geographical distribution as well as morphological variability of the genus. Determination of the material was done mostly from living flowering material by JL-Š. One yet undescribed species, was used in this paper. Its preliminary working name has been indicated with a specific epithet starting with a capital letter in the phylogenetic trees. Elsewhere in the paper, this epithet is in parentheses and indicated as “ined.” Orchidantha calcarea M.R.Hend. has been upheld at specific rank due to some reasons. Based on our study of living flowering material, we also consider O. longisepala D.Fang to be a distinct species rather than a variety of O. chinensis.
The outgroups were selected to cover five major lineages in Zingiberales (one sample from each of the three other families in the “banana group,” and one sample from each of the two major lineages in the “ginger group,” represented by Marantaceae and Zingiberaceae). To root the order, we used a single sample from the sister order (Hanguanaceae, Commelinales).
Genome Size and Chromosome Counts
To explore the evolution of genome size in the family, we measured DNA content and counted chromosomes for the samples. Holoploid genome sizes, equivalent to nuclear DNA 2C-values, were estimated using propidium iodide flow cytometry (FCM). Sample preparation generally followed the simplified procedure originally described by Otto et al. (1990). About 1 cm2 of young and intact fresh leaf tissue and an internal standard were co-chopped in a sandwich-like arrangement with a sharp razor blade in 0.5 ml of ice-cold Otto I buffer (0.1 M citric acid, 0.5% Tween 20). The nuclear suspension was filtered through a nylon mesh with a 42-mm pore size. After incubating for 10 min at room temperature, 1 mL of Otto II buffer (0.4 M Na2HPO4⋅12H2O), supplemented with propidium iodide at a final concentration 50 μL⋅mL-1, RNase IIA (50 μL⋅mL–1) and 2-mercaptoethanol (2 μL⋅mL–1), was added. The samples were incubated for 10 min at room temperature, after which a fluorescence intensity of 3,500 particles was recorded on a Partec Cyflow instrument (Partec GmbH, Münster, Germany) equipped with a 532-nm solid-state laser (Cobolt Samba 100 mW, Cobolt, Sweden). Each plant was re-analyzed at least three times on different days and only histograms with peaks of approximately the same height were accepted. If between-day variation (max./min. value) exceeded 2%, the outlying value was discarded and the sample re-measured. Bellis perennis L. (2C = 3.42 pg) was selected as a primary internal reference standard for all species. The genome size of the primary standard has been established by calibration against Pisum sativum with 2C = 8.76 pg (Greilhuber et al., 2007; based on 14 replications). In total, 38 samples were measured.
Chromosomes for 24 accessions belonging to 16 species were counted. Actively growing, thick roots (1–2 mm in length, 1–3 mm in diam.) were collected from living plants at Singapore Botanic Gardens in the morning (9–10 a.m.). The roots were kept in tap water in an ice bath in the dark for 24 h, after which they were fixed in Farmer’s solution (1 part glacial acetic acid, 3 parts 99% ethanol) for 0.5–10 h. If stored for longer, the roots were transferred to 70% ethanol. After fixing, the roots were dried with tissue paper and transferred to soften in an enzyme buffer (40 mM citric acid, 60 mM tri-sodium citrate dihydrate), pH 4.8 (Setiawan et al., 2018), with 4% cellulase from Aspergillus niger and 4% commercial pectic enzyme (pectinase and maltodextrin, unspecified ratio). Softening was done for 1–2 h at 37°C. After softening, c. 1 mm of the tip of the root was cut and stained in 30 μL lactopropionic orcein (2 g orcein powder, 50 mL lactic acid, 50 mL propionic acid, 122 mL ddH2O, combined then filtered through a 40 μm cell strainer) for 5 min. The tip was then moved to a glass slide together with the lactopropionic orcein, covered with a cover slip, avoiding bubble formation, and gently tapped with a pencil 20–50 times, until all visible pieces of tissue were fully dispersed. Larger root tips were divided over several slides. The cover slip was firmly pressed with two thumbs for 60 s. Metaphase cells were photographed under 400 × magnification with an oil drop.
Bait Design
To generate sequences for phylogenetic reconstruction, we employed HybSeq, a cost-effective and increasingly popular target enrichment approach that uses baits based on known sequences to enrich sequencing libraries for hundreds to thousands of target loci (Faircloth et al., 2012; Mandel et al., 2014; Weitemier et al., 2014). Bait design was carried out using Sondovač 1.3 (Schmickl et al., 2016) using recommended settings for all parts. Part A of the pipeline was modified to correct a typographic error (two occurrences of “$FASTQNOCP’_” were changed to “$FASTQNOCP’.” on line 1171 of file sondovac_part_a.sh), and new lines were added to separate the sequence name and sequence in the fasta output of sondovac_part_a.sh). As a reference, we used the transcriptome assembly of O. maxillarioides from OneKP Major Release (ERS1829820, Leebens-Mack et al., 2019) by using the file LSKK-SOAPdenovo-Trans-assembly.fa.bz2 available at http://www.onekp.com/public_data.html. The closest available whole genome Illumina library, Musa acuminata subsp. acuminata (SRX3579252), was used to filter repetitive elements. The transcriptome assemblies were filtered by removing any contig pairs with > 90% similarity, taken as an indication of multicopy genes. Reads matching the published plastome of O. fimbriata (KF601569) were removed, and assemblies with > 1000 BLAST (Kent, 2002) hits using Musa reads were excluded. Contigs shorter than 120 bp were excluded. For exon filtering, a minimum gene length of 600 bp was used, but the pipeline failed to find complete CDSs at this length, likely due to incomplete assemblies, and the target regions therefore included all contigs over 120 bp long. The final target regions were filtered to remove all sequences with more than four consecutive unknown nucleotides. The final number of targets was 3,333 sequences with a total length of 987,972 bp. A custom bait set was designed by MyBaits (MYcroarray, United States), based on the target regions. The baits were 120 bp long, with 2 × tiling. The total number of baits was 18,283.
DNA Extraction and Sequencing
DNA was extracted using a modified CTAB method (Doyle and Doyle, 1987). Total genomic DNA was sheared to an average fragment length of 300 bp using a Covaris ME-220 focused-ultrasonicator (Covaris Inc., Woburn, MA, United States). DNA libraries were constructed using the NEBNext Ultra II DNA Library Prep Kit for Illumina with unique dual index adapters (New England Biolabs, Singapore) with 400 μL total genomic DNA. Size selection was carried out to an average fragment length of 300 bp. The number of PCR cycles varied from 6 to 9 (median 6), and if the concentration was low, further PCR cycles were carried out, to a cumulative total of 9 to 16 cycles (median 13). Enrichment of the libraries for the target loci was carried out using the custom MyBaits kit, following the recommended temperature and time for hybridization (65°C for 16 h). The number of PCR cycles after hybridization varied from 9 to 14 (median 12).
Sequencing was carried out by Novogene (Singapore) using Illumina HiSeq, using paired-end sequencing with a read length of 150 bp.
Sequence Assembly and Phylogenetic Reconstruction
We trimmed reads using Trimmomatic (ILLUMINACLIP: TruSeq3-PE.fa:2:30:10 HEADCROP:3 LEADING:30 TRAILING:25 SLIDINGWINDOW:4:25 MINLEN:20) (Bolger et al., 2014). Trimmed reads were assembled using HybPiper, which produces gene-by-gene, de novo assemblies guided by a set of reference CDSs (Johnson et al., 2016). Assemblies were carried out using default parameters, with the transcriptome reads used for bait design as the reference. For further analyses, we used the default HybPiper output, which is the predicted CDS for each gene.
We filtered sequences to avoid artifacts caused by excessive missing data. The average length of each gene was calculated, and sequences less than 25% of this length were discarded, as were genes containing sequences for less than 20 samples. We aligned each gene using MAFFT (Katoh and Standley, 2013) (default parameters) and used TrimAl (Capella-Gutiérrez et al., 2009) to discard sequences with an average pairwise identity of less than 0.5 to all other sequences in the alignment (indicative of a poor quality sequence that could not be properly aligned) as well as columns containing more than 75% gaps. We used RAxML 8.2.4 (Stamatakis, 2014) to estimate a maximum-likelihood tree for each gene individually (GTRCAT model, 200 bootstrap replicates). We then used ASTRAL-III (Zhang et al., 2018), a summary-coalescent method, to estimate a species tree from all gene trees, estimating node support using local posterior probability (LPP, default) as well as quartet scores (-t 1) representing gene tree concordance. We also used ASTRAL-III to test whether, for each node, the null hypothesis of a polytomy could be rejected (null hypothesis of polytomy was not rejected if p > 0.05, Sayyari and Mirarab, 2018). We also used RAxML to estimate two maximum-likelihood trees for a partitioned supermatrix of all genes (supermatrix). Alignment, trimming and estimation of gene trees were parallelized using GNU Parallel (Tange, 2018). For most analyses, we dropped superfluous samples to retain only one sample per species (singleton tree), choosing the sample with most genes available.
Divergence Time Estimation
We estimated divergence times on the singleton tree under penalized likelihood using the “chronos” function in ape 5.3 (Paradis and Schliep, 2019) in R (R Development Core Team, 2019). The smoothing parameter (λ) was determined using the cross-validation method (Sanderson, 2002) and testing values from 10–5 through 1015. The tree was time calibrated under three clock models: discrete, correlated, and relaxed, and the best-fit model was chosen using the Phylogenetic Information Criterion (ΦIC) scores (Paradis, 2013). Internal fossils are not available for Lowiaceae. Therefore, for dating our phylogenies, we used node ages provided for the order by Harris and Davies (2016), who paid particularly careful attention to the placement of fossils in Zingiberales; the dates are broadly similar to those provided by Kress and Specht (2006). We fixed the stem age of Lowiaceae (crown of Lowiaceae + Streliziaceae) at 61.45 million years ago (MYA), and the crown age of Zingiberales at 93.73 MYA; all the dates from our analyses should be considered minimum ages. As the penalized likelihood approach used does not integrate over model uncertainty or uncertainty in calibration placement and timing, confidence intervals on node ages are not provided in this study. However, resulting trees were also visualized using the densiTree function in phangorn 2.4.0 (Schliep, 2011) to assess the sensitivity of the three models to changes in λ. A geologic timescale based on the strat2012 dataset was added to tree figures using PHYLOCH 1.5-3 (Heibl, 2008 onward). As an independent analysis, we also estimated the divergence times using treePL with the same fossil calibration points (Smith and O’Meara, 2012).
Ancestral Character State Reconstructions
All Lowiaceae species involved in the study were scrutinized for critical morphological characters. A total of 33 informative characters covering both vegetative and floral morphology were scored from information provided in original descriptions, supplemented by our measurements from the living flowering material in the field and living collections at Singapore Botanic Gardens, Sabah Agriculture Park (Tenom), Kipandi Butterfly Park as well as spirit material available at SING. Nineteen characters were scored as discrete traits, while the remaining 14 were quantitative and scored as mean values (Supplementary Table 2). To visualize these characters in a phylogenetic context, a non-metric multidimensional scaling (NMDS) ordination was generated using the metaMDS function in the vegan package in R (Oksanen et al., 2020). The first two axes were then plotted using the phylomorphospace function in phytools. Due to the lack of overlap in morphological characters, outgroups were not included in these analyses.
Ancestral character state reconstruction was carried out using the fitER function in phytools 0.7–70 (Revell, 2012) in R on the ingroup-only time-calibrated tree. Three models were tested (“ER,” “SYM,” and “ARD”), and the best-fit model was chosen based on AIC. Characters were mapped to the singleton tree either as categorical or continuous characters. The flow-cytometry results were adjusted to 1Cx and reconstructed as a continuous character using stochastic mapping as implemented in the make.simmap function in phytools. The results were visualized with 95% confidence intervals using the phenogram95 function.
Ancestral Range Reconstruction
Ancestral ranges were estimated under the DEC and DEC + J models as implemented in BioGeoBEARS 1.1.1 (Matzke, 2013), using the time-calibrated tree with outgroups removed. Species distributions were coded using three areas: Borneo, Thai-Malay Peninsula (up to the Isthmus of Kra), and Indochina (including the rest of Thailand and South China). Areas were determined based on a review of relevant literature, including all relevant protologues, as well as specimens preserved at E, K, L, P, SAN, SING. The analysis was run under default parameters, allowing up to two simultaneous areas per node.
Results
Chromosome Counts, Ploidy Levels and Genomes Size
Flow-cytometric analyses yielded high-resolution histograms (Supplementary Figure 1). The coefficients of variation (CVs) of G0/G1 peaks ranged from 1.25 to 4.34% (median 2.15%) for the Lowiaceae samples and from 1.38 to 4.06% (median 2.21%) for the reference standard. Day-to-day fluctuations caused by instrument instability or differences in sample preparation were negligible; the standard error of mean of repeated measurements on different days ranged between 0.05 and 0.5%. The absolute genome sizes varied in our data set from 2C = 4.632 pg in O. gigantea to 2C = 15.260 pg in O. chinensis, representing a 3.29-fold range. However, the 1Cx values showed a much smaller variation within the genus, ranging from 1Cx = 2.316 pg in O. gigantea to 1Cx = 3.481 pg in O. calcarea, representing only a 1.5-fold range (Figure 4 and Supplementary Table 3).
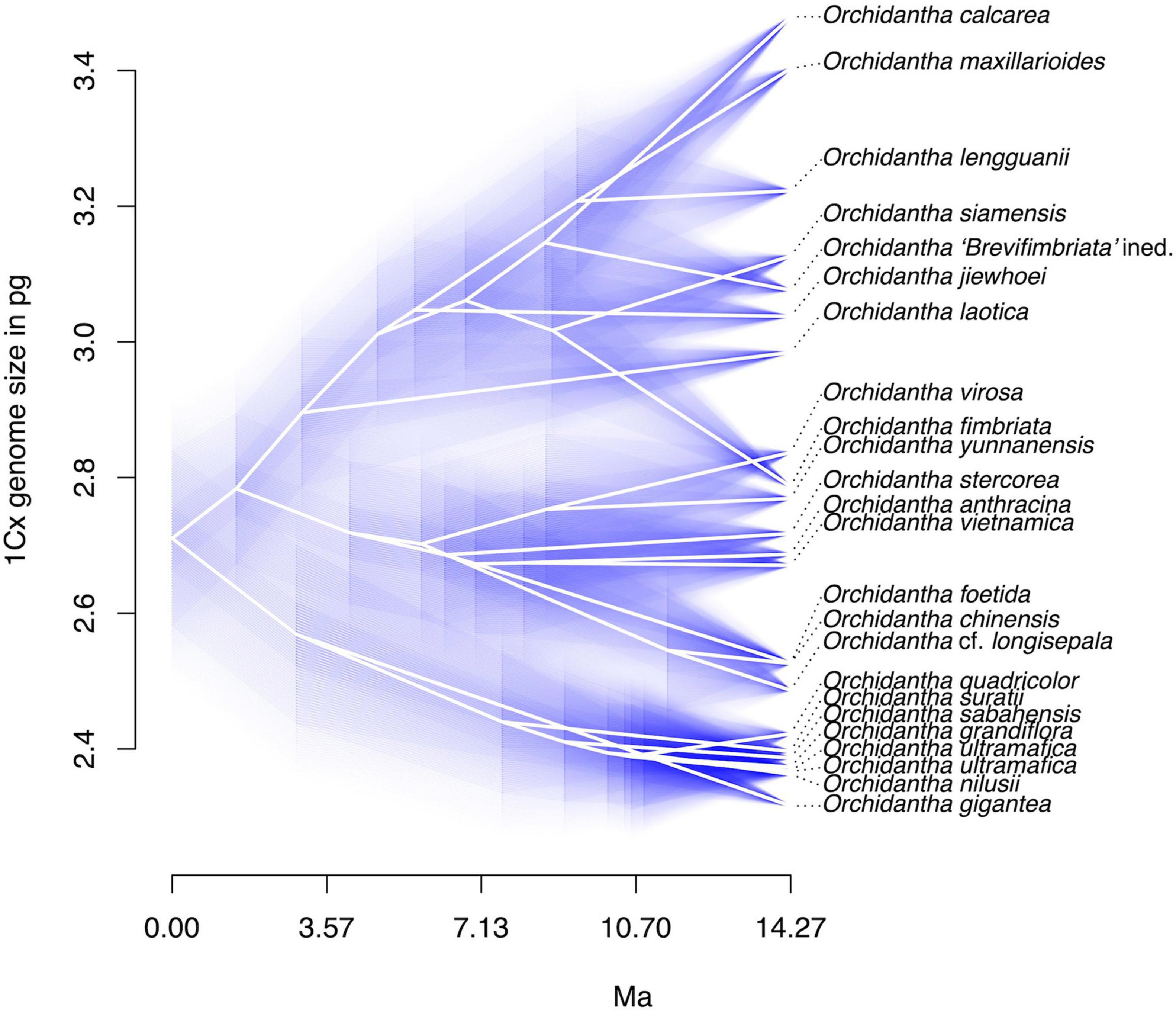
Figure 4. Monoploid (1Cx) genome sizes in Orchidantha in picograms, displayed in a phenogram with confidence values for genome size.
For eight species, the genome sizes were measured for more than one accession. The intraspecific variability (based on 1Cx) varied from 0.1 to 2.3% in most species; it was higher in O. siamensis (4.5%) and O. fimbriata (6.4%). The slightly elevated variation in these two species is not surprising given their wider distribution and a level of morphological variability that suggests the existence of possible cryptic species in these two taxa. Similar variation was observed in O. laotica K.Larsen (5.8%), when one sample of uncertain identity was included (the variation decreased to 2.3% when this sample was excluded).
Orchidantha anthracina H.Ð.Trần, Luu and Škorničk., O. “Brevifimbriata” ined., O. calcarea, O. foetida T.Jenjittikul and K.Larsen, O. grandiflora, O. laotica, O. quadricolor, O. siamensis, O. stercorea H.Ð.Trần and Škorničk., O. ultramafica Škorničk. and A.Lamb, O. vietnamica K.Larsen, O. virosa and two accessions of O. fimbriata (JLS-1893, JLS-3159) were 2n = 18. Orchidantha lengguanii Škorničk. and two accessions of O. fimbriata (JLS-3162 and JLS-914), were 2n = 27 (Supplementary Figure 2). Orchidantha chinensis and O. cf. longisepala D.Fang were both 2n = 54.
Phylogenetic Analyses
After filtering, the supermatrix contained 48 samples and 3,101 loci. It had 925,793 characters and 124,155 parsimony-informative sites, with 8.32% gaps and undetermined characters.
Both phylogenetic analyses strongly support the monophyly of Lowiaceae (Figure 5, 100% RAxML bootstrap support, 100 LPP, polytomy test p = 0), and the genus is likely most closely related to Strelitziaceae (100 BS, 100 LPP, polytomy test p = 0). In both analyses, the Bornean species other than O. jiewhoei form a clade (here referred to as the “Borneo clade”; 100 BS, 100 LPP, polytomy test p = 0). The Vietnamese and Chinese species form a clade (“Indochina clade”; 100 BS, 100 LPP, polytomy test p = 0) and are sister to other continental Asian species plus O. jiewhoei (“widespread clade”; 100 BS, 100 LPP, polytomy test p = 0); together these two clades form the Indochina + widespread clade, sister to the Borneo clade (100 BS, 94 LPP, polytomy test p = 0.02). In the widespread clade, there are three subclades: accessions of O. laotica form a clade (“Laotica subclade”; 100 BS, 100 LPP, polytomy test p = 0) that is sister to the other two (100 BS, 100 LPP, polytomy test p = 0); Orchidantha jiewhoei (Borneo), O. maxillarioides and O. lengguanii (both Peninsular Malaysia) form a clade that is morphologically and geographically more diverse (“Maxillarioides subclade”; 100 BS, 100 LPP, polytomy test p = 0), and the morphologically and geographically similar white labellum species form a clade (“Fimbriata subclade”; 100 BS, 100 LPP, polytomy test p = 0).
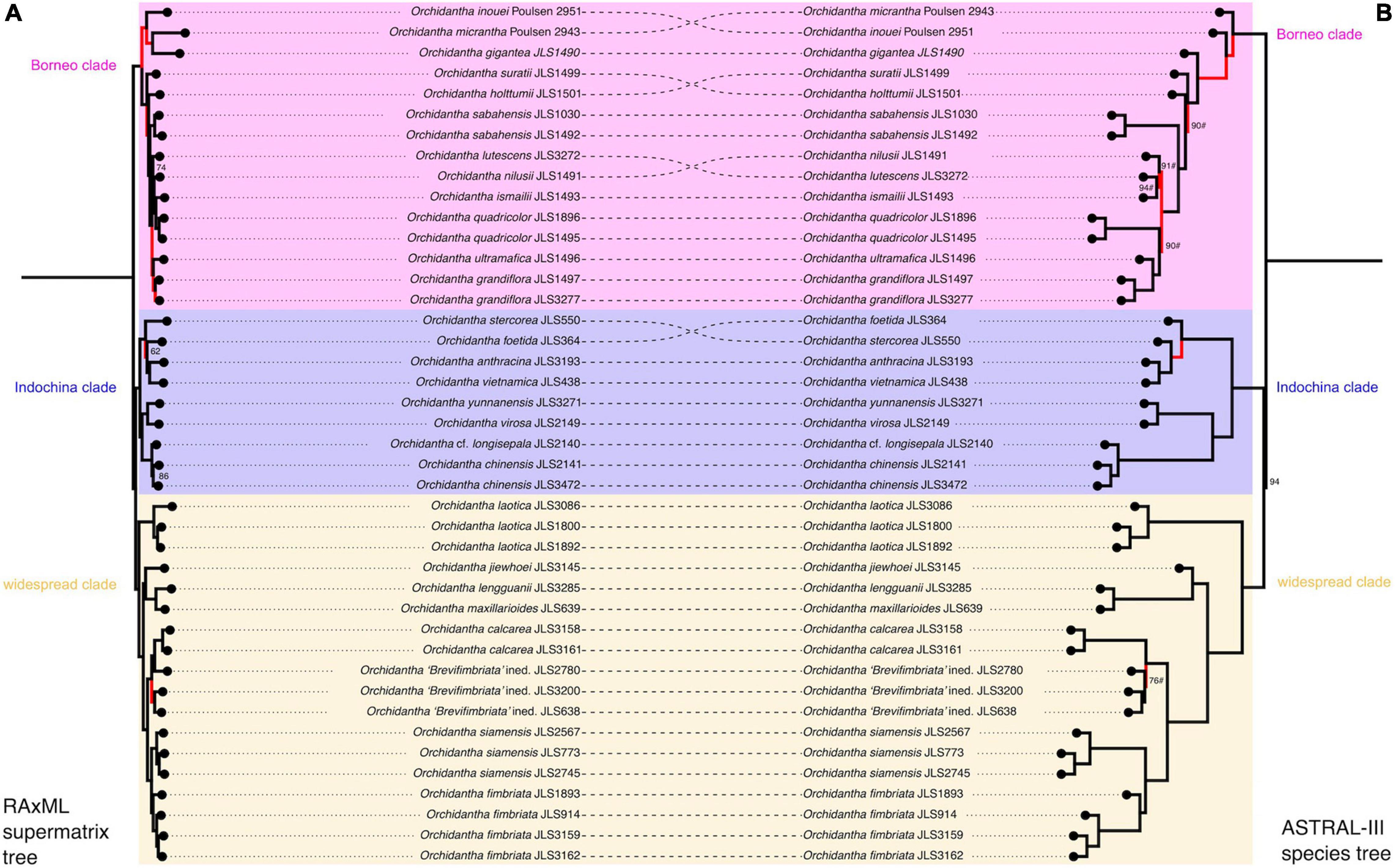
Figure 5. Phylogenetic analyses of the supermatrix dataset, which includes all samples in this study. (A) Maximum likelihood analysis of a concatenated sequence supermatrix with RAxML bootstrap support. (B) ASTRAL species tree computed from individual gene trees with ASTRAL quartet score. Conflicting parts of the topologies are highlighted in red. The background colors refer to the three clades: pink = Borneo clade; blue = Indochina clade; yellow = widespread clade.
The topology of the maximum likelihood tree based on a concatenated supermatrix and the ASTRAL species tree based on the 3,101 gene trees differed in four places only. The conflicting branches are highlighted in red in Figure 5. The above analyses were conducted using the full supermatrix dataset; fourteen duplicate samples were dropped from the singleton tree (JLS2780, JLS3200, JLS3158, JLS3086, JLS1030, JLS2141, JLS1893, JLS3159, JLS914, JLS3277, JLS1892, JLS1896, JLS2567, and JLS2745). Orchidantha “Brevifimbriata” ined. was not monophyletic in the supermatrix analysis (Figure 5). The lowest quality sample (JLS2780) was closer to O. calcarea in the analyses than the other two samples.
Crown ages for Lowiaceae and its major sub-clades appear in Table 1. The discrete clock model was the best fit under ΦIC, although we acknowledge that the likelihood scores between that model and the relaxed or correlated models may not be directly comparable (Paradis, 2013), and we therefore also report ages for the second-best fit model (correlated). The results from the discrete clock model were also closest to those derived from treePL, differing by no more than 0.19 MY for any node (Supplementary Figure 3). The crown age of Lowiaceae under the discrete model was 14.27 MYA. The Borneo clade had a minimum crown age of 11.4 MYA, the Indochina clade 10.16 MYA, and the widespread clade 11.27 MYA (Supplementary Figure 4). Using a correlated model, the ages were strongly affected by the value of λ (rate smoothing parameter), and the crown age of Lowiaceae varied from 46.09 MYA at λ = 0.1 to 25.57 MYA at λ = 100 (Supplementary Figure 5).
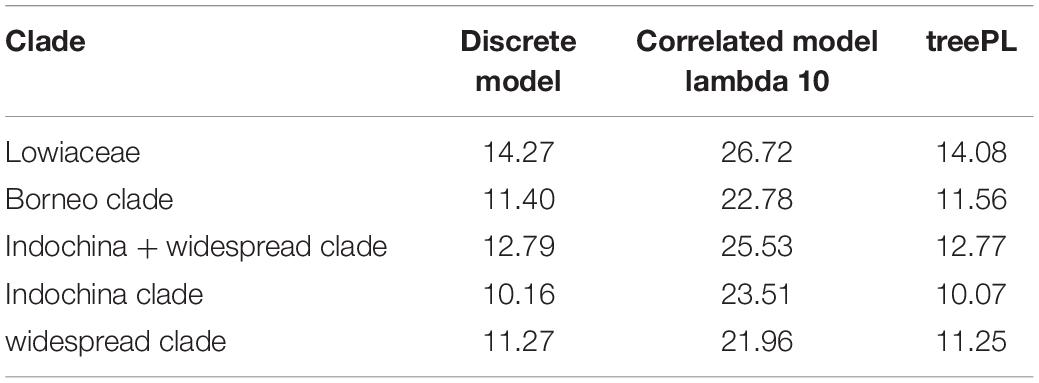
Table 1. Crown ages under three time-calibration schemes: the discrete model in chronos, the correlated clock model in chronos, and treePL.
Character Evolution
The NMDS analysis shows weak spatial segregation between major clades, with all clades considerably overlapping in all characters (Figure 6). The overlap remains when quantitative and categorical characters are separated (Supplementary Figures 6, 7).
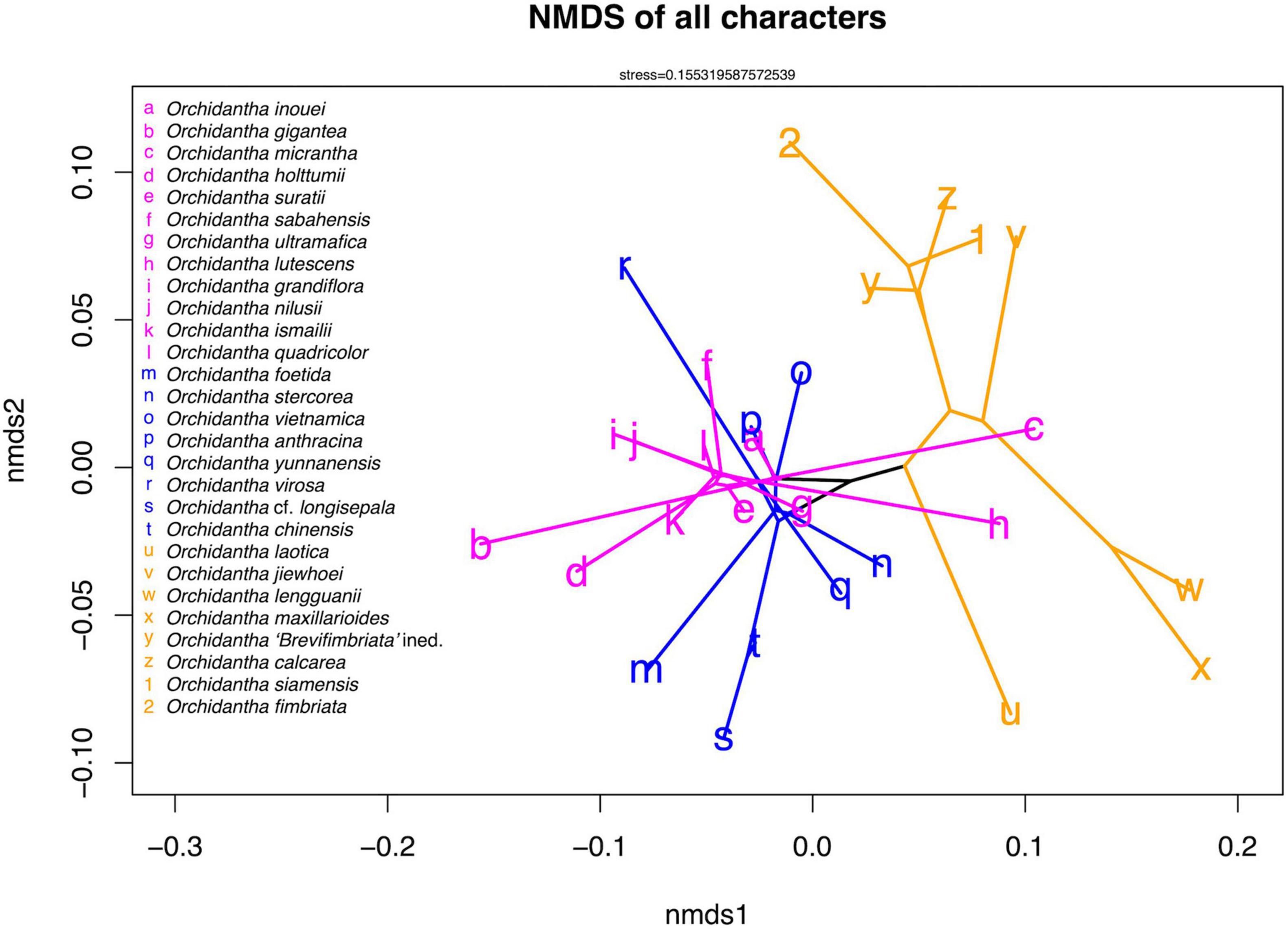
Figure 6. Non-metric multidimensional scaling (NMDS) ordination of all scored non-genomic characters of Orchidantha. The ordination was generated using the metaMDS function in the vegan package in R, with the first two axes plotted using the phylomorphospace function in the phytools package in R. The clade colors follow Figure 5.
In the character state reconstruction, the ancestor of the genus most likely had petiolate leaves; cream-white to yellow-green, unequally sized, untwisted sepals, with a flat dorsal sepal arching over the labellum and lateral sepals that support the labellum (claw-type flower); a clawless, entire, dark-purple (black-flower type) labellum without slimy exudates or a prominent midrib (the midrib extended to a small apicule); irregularly elliptic to rectangular, dark-purple, flat petals that covered the stigma; stigma denticulate to serrulate; a heart-shaped viscidium; and a fecal to decomposing smell (Supplementary Figures 8–42).
Biogeography
There is a strong geographic pattern to the phylogeny of Lowiaceae: Indochinese species are from one clade (Indochina clade), except for O. laotica. Species from Borneo are from one clade (Borneo clade) except for O. jiewhoei. The widespread clade is mostly present in the Thai-Malay Peninsula, with the exception of O. laotica and O. jiewhoei (Figure 7).
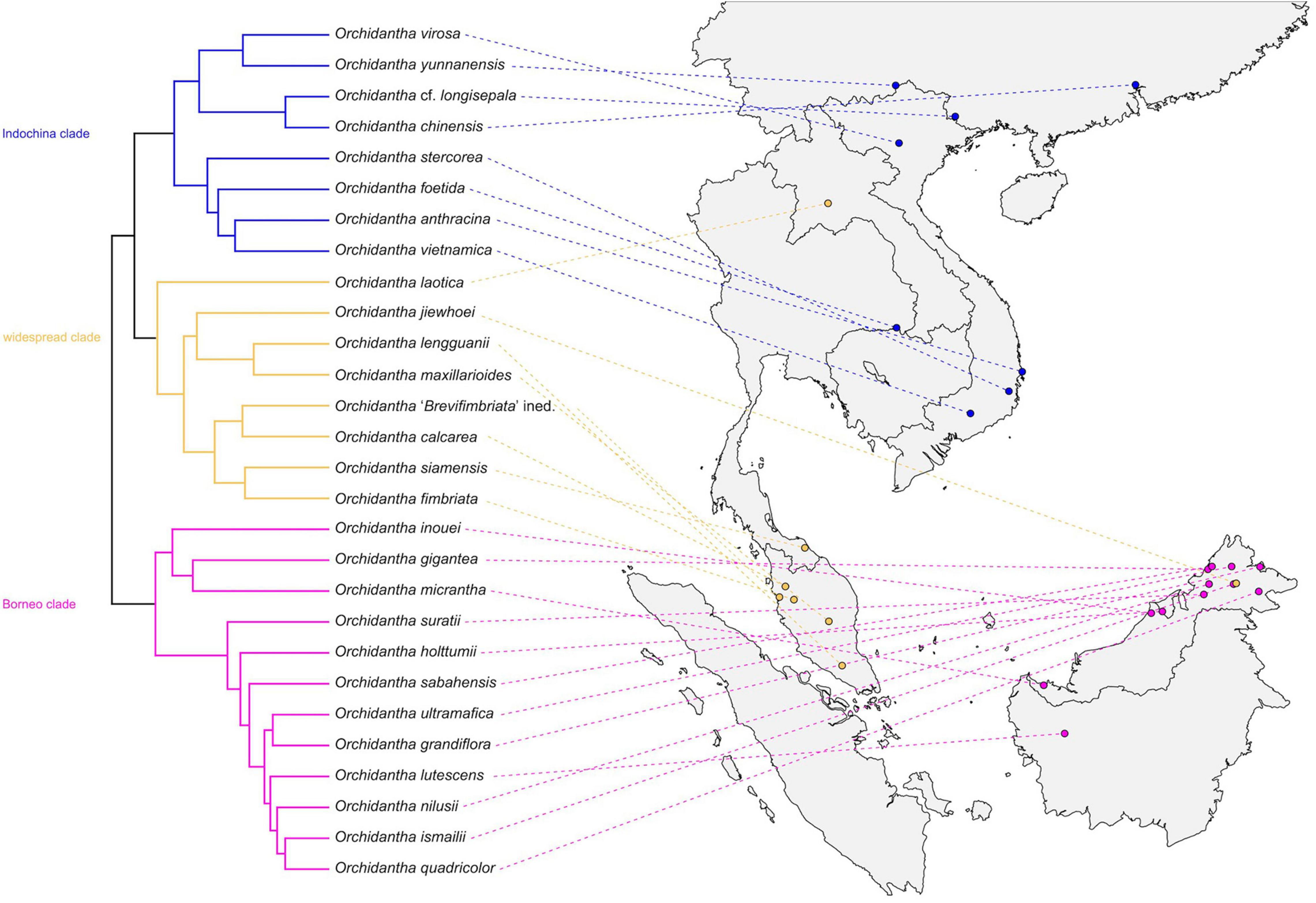
Figure 7. Distribution of the Orchidantha species in this study linked to a phylogenetic reconstruction with one sample per species. The clade colors follow Figure 5.
The DEC analysis of BioGeoBEARS places the crown node of Orchidantha in Borneo and Indochina. The stem and crown nodes of the Borneo clade are placed in Borneo. The stem and crown nodes (MRCA) of the Indochina + widespread clade are placed in Borneo and Indochina. The stem and node of the Indochina clade are placed in Indochina only. The stem and crown nodes of the widespread clade are placed in Borneo and Indochina, from where it dispersed more widely, with the Laotica sublade placed in Indochina, the Maxillarioides subclade placed in Borneo and the Thai-Malay Peninsula (later splitting to the two regions reflecting their current distribution), and the Fimbriata subclade placed entirely in the Thai-Malay Peninsula (Figure 8). In the DEC + J analysis, the crown node of Orchidantha was also in Borneo and Indochina, but the crown of the Indochina + widespread clade was in Indochina, with a later dispersal to Peninsular Malaysia and again to Borneo (Supplementary Figure 43). The likelihoods of the two analyses were not compared as they are not comparable (Ree and Sanmartín, 2018).
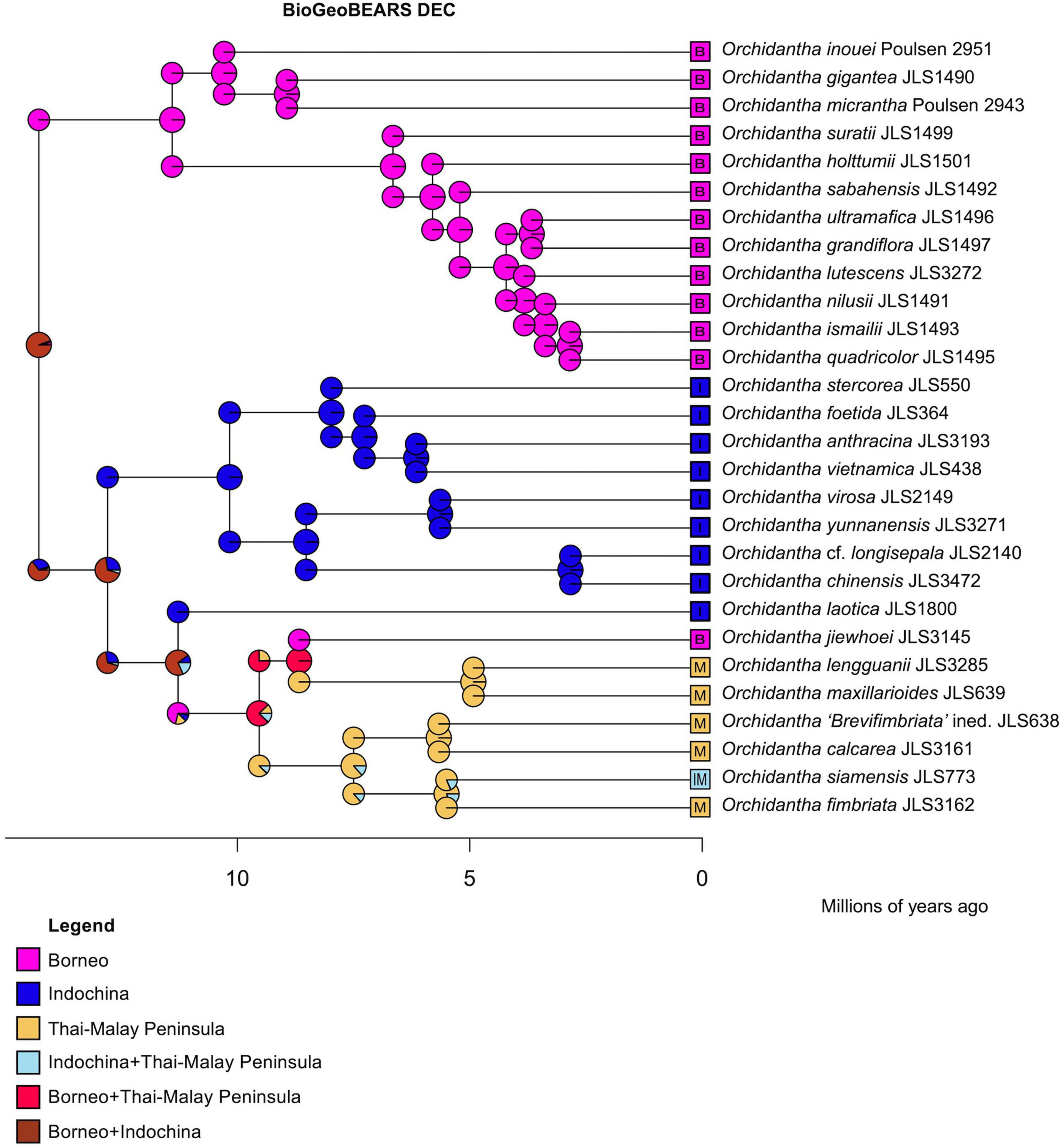
Figure 8. Biogeographical analysis using DEC in BioGeoBEARS using the globally optimum parameter values estimated by ML, with all nodes limited to be present in two areas, maximum.
Discussion
Genomes Size, Chromosome Numbers and Ploidy Levels
Orchidantha maxillarioides, 2C = 6.86 pg (Šmarda et al., 2014) and 2C = 7.10 pg (Bharathan et al., 1994) was previously the only member of the family with a published genome size. Both reported values are nearly identical to our estimation for this species, differing from them by only 0.7 and 4.2%, respectively. This difference is fully within the variation range between different laboratories caused by methodological constraints (Doležel et al., 1998). Genome sizes of Orchidantha species are classified as being intermediate (all samples but that of hexaploid O. chinensis and O. longisepala) or large (two hexaploid species) and all are higher than the median value for vascular plants (2C = 3.36 pg, Leitch et al., 2019). Orchidantha genome sizes (GS) are also on the upper end of the GS range of the whole order. The ecological significance of genome sizes remains obscure. In angiosperms, genome size is positively correlated with specific leaf area (Orchidantha have a high specific leaf area and large genomes), but this distinction is driven by recent polyploidy, is not observed within closely related taxonomic groups, and seems to not be correlated with metabolic rates; also, the trend is opposite in gymnosperms (Knight et al., 2005; Morgan and Westoby, 2005; Beaulieu et al., 2007).
Our chromosome counts agree with previous studies that have reported the basic chromosome count in Lowiaceae to be 1x = 9, and we therefore consider species with a chromosome count of 2n = 18 to be diploid. Our results indicate that most Orchidantha species are diploids. The only polyploid taxa previously reported are two hexaploid species O. chinensis and O. longisepala (Song et al., 2003, 2004); these counts were confirmed in our study. Two out of the four samples of O. fimbriata, and the only sample of O. lengguanii included in our study, are triploids (2n = 27). The discovery of triploid samples in our dataset is unusual. Triploid individuals cannot usually reproduce sexually, and are therefore rare in the wild. In some cases, such as in another Asian forest herb genus, Hanguana (Niissalo et al., 2020), commonly occurring triploidy can be explained by apomixis, but we have not observed fruit set in the triploid Orchidantha plants, and we do not suspect that these samples could be apomictic clones. Triploid plants could also possibly be hybrids between tetraploid and diploid plants, but no tetraploid species are known in Orchidantha, as already pointed out by Song et al. (2004). It is likely that the triploid samples represent aberrant individuals, though this should be confirmed by wider sampling from both O. lengguanii and O. fimbriata. Triploid individuals occur occasionally in otherwise diploid populations due to unreduced gametophytes, from fertilization of an egg with two sperms. We conclude that Orchidantha has relatively consistent genome sizes, few polyploid taxa and a consistent basic chromosome number.
Phylogenetic Relationships and Character Evolution
We find strong support for a sister relationship between Strelitziaceae and Lowiaceae. This relationship has been consistent in previous analyses (Carlsen et al., 2018). Our results can be taken as further support for this relationship. However, the relationships of the other outgroup families included may be affected by limited sampling within them. Within Orchidantha, the overall topologies of our phylogenetic reconstructions are similar to those observed by Johansen (2005), with the Bornean species being sister to the rest of the genus. Despite our wider sampling, there is a strong geographic pattern to the phylogenies. A more detailed comparison of our results to Johansen’s is complicated by different taxonomic sampling, and the uncertainty of the identity of a few samples in Johansen’s study (particularly the samples of O. borneensis N.E.Brown, O. fimbriata and O. longiflora included therein). In our Astral reconstruction, the Sarawak samples were sister to the rest of Bornean material, as in Johansen (2005), but the support for this relationship is relatively low and it is not observed in the supermatrix tree.
The node ages derived from the discrete model in chronos can be taken as reasonable minimum ages for nodes in Orchidantha. The values reported by the discrete model in chronos and by treePL were clearly younger than those observed when using a correlated model in chronos. The latter, however, was very much affected by the selection of lambda. For the calibration of our tree, we rely entirely on secondary calibration points. Also the stem leading to Lowiaceae is long with no branches after the divergence from Strelitziaceae. It is perhaps not surprising that the different analyses give such different dates; however, from the perspective of reconstructing the geographic history of Orchidantha, there are no big differences between the different results. In the discussion, we assume that the dates from the discrete model analyses are approximately correct.
Our phylogenetic reconstructions show that Orchidantha can be divided into several well-supported clades based on genetic data. However, these clades are more difficult to characterize morphologically. Species with distinct flower types, either by the color of the labellum (black, white and puce) or flower shape (claw-like or propeller-type flowers) do not form entirely distinct lineages; mostly black-labellum species with claw-like flowers form a grade in which a clade of pale-flower species with propeller-type flowers is embedded.
There are three main lineages of black-flowered species: the Borneo clade, the Indochina clade and the Laotica subclade. These three lineages are morphologically similar to each other, with no consistent characters to separate them (Figure 9). There are local patterns particularly in the evolution of flower color, shape of the perianth parts and viscidium morphology, but these do not aid in the separation of the major lineages of the black-flowered species, and there is considerable morphological overlap between the clades seen in the NMDS analyses of all morphological data types (Figure 6). No living species shares the exact set of categorical characters that was assigned to the basal node of the genus in the ancestral state reconstruction (Supplementary Figures 8–27), but the proposed ancestral form has many similarities to the Borneo and Indochina clades and Laotica subclade of the widespread clade. The puce and white labella, propeller-type flowers and mushroom smell, all common in Maxillarioides and Fimbriata subclades of the widespread clade, are likely to be derived character states.
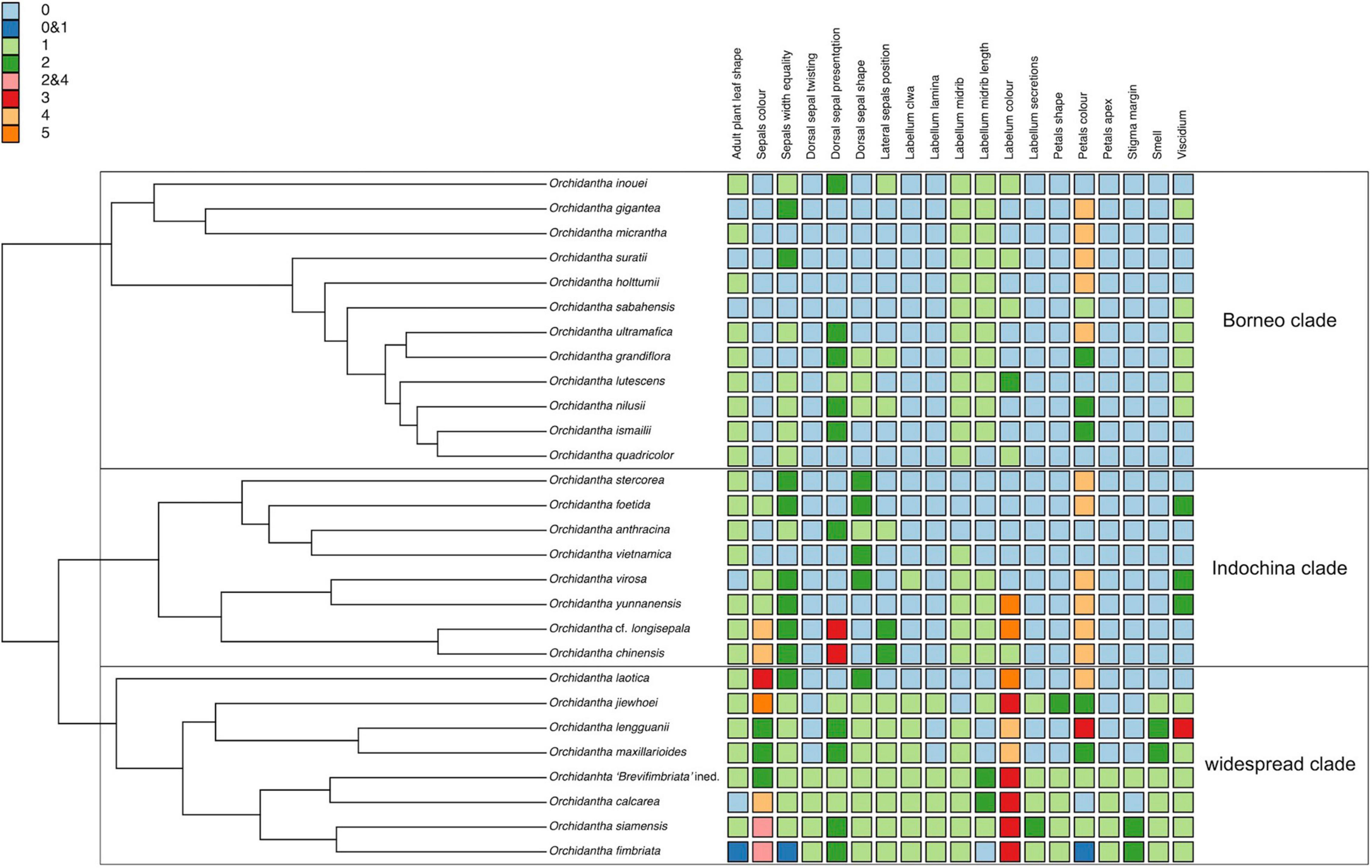
Figure 9. Categorical morphological characters on Orchidantha species mapped to a phylogenetic reconstruction with one sample per species. Adult plant leaf shape 0: gradually tapering without prominent petiole; 1: prominently petiolate. Sepals color 0: Cream-white to yellow-green (or with pale tinge externally); 1: Yellow-green to dull green internally with rich purple tinge externally; 2: Reddish-purple base, greenish apex; 3: Pale green base, reddish-purple-maroon apex; 4: Purple or maroon; 5: Solid bright green throughout. Sepal width equality 0: Equal; 1: Dorsal narrower; 2: Dorsal broader. Dorsal sepal twisting 0: Straight; 1: twisting. Dorsal sepal presentation 0: Arching over labellum; 1: Upright, or slightly reflexed not arching; 2: Strongly reflexed; 3: Front facing. Dorsal sepal shape 0: Flat with margins flat; 1: Margins reflexed; 2: Concave (boat-shaped). Lateral sepals position 0: Supporting labellum; 1: Spreading like a propeller and not supporting labellum; 2: Forward facing, slightly spreading like a propeller and not supporting the labellum. Labellum claw 0: Absent; 1: Present. Labellum lamina 0: Entire; 1: Lobed. Labellum midrib 0: Not prominent; 1: Prominent. Labellum midrib length: not extending to apicule; 1: Extending to a small apicule; 2: Extending to prominent apicule. Labellum color 0: Dark purple; 1: Purple, but yellow or white distally; 2: Yellow with slight purple patch at base; 3: White with some purple at claw; 4: Dirty pinkish to brown; 5: Dark purple with greenish to yellow midrib/central area. Labellum secretions 0: Not slimy; 1: Slightly slimy especially with age; 2: Prominently slimy. Petals shape 0: Irregularly elliptic to rectangular; 1: Narrowly triangular; 2: Regularly elliptic. Petals color 0: Cream-white, sometimes with very slight purple tinge; 1: Cream-white, purple at margins apically; 2: Cream-white at base, purple at apex; 3: Purple with greenish apex; 4: Purple to dark purple throughout. Petals apex 0: Flat, covering all or most part of stigma; 1: Reflexed (usually at least partly exposing stigma, but not in O. calcarea, where the stigma remains hidden). Stigma margin 0: denticulate to serrulate; 1: Shortly fimbriate; 2: Long fimbriate. Smell 0: fecal or decomposing; 1: Sweaty or rancid; 2: Faint mushroom. Viscidium 0: Heart-shaped; 1: V-shaped; 2: Y-shaped; 3: broadly U-shaped.
Species with white and puce labella, combined, are monophyletic within the widespread clade. They form two of the three subclades within the widespread clade: the Maxillarioides subclade and the Fimbriata subclade (Figure 3). Combined, they are characterized by a broad, flat labellum with a basal claw, propeller-type flowers, and by the absence of a strong smell of decaying meat or feces. Outside this clade, the basal claw is only known in O. virosa, and the propeller-type flowers are known in O. anthracina, O. grandiflora, and O. nilusii Škorničk. and A.Lamb. In gross flower morphology, these species are clearly different due to their dark, clawless labella; they also all have strongly smelling flowers. The species in the Fimbriata subclade are most obviously characterized by their reflexed petals. This character is absent in the Maxillarioides subclade and in the rest of the genus.
As the main lineages of Orchidantha can’t be morphologically defined, we refrain from proposing a formal subgeneric classification here. Informal clade names can be used when necessary (Indochina clade, Borneo clade, widespread clade, Laotica subclade, Maxillarioides subclade and Fimbriata subclade); of these, only the Fimbriata subclade is clearly morphologically defined.
Biogeography
The geographic history of the family could be entirely explained by vicariance and gradual dispersal along landmasses; the divergence dates derived from the DEC analysis agree with land mass distribution in the range of the family. Analysis using DEC + J resulted in a different scenario, with initial distribution ranging from Borneo to Indonesia, and later dispersal to Peninsular Malaysia and back to Borneo. However, the use of DEC + J has been heavily criticized as it is likely to overestimate the number of dispersal events (Ree and Sanmartín, 2018). If the scenario suggested by DEC is true, the ancestral distribution area of the genus ranged from Borneo to Indochina (what was then north-eastern Sundaland). From there, the Indochina and Borneo clades diversified in geographically distinct areas, whereas the widespread clade only later divided geographically to the regions where it is now found: Indochina, Borneo and Peninsular Malaysia. The ancestral area of north-eastern Sundaland recovered in our DEC analysis is similar to the findings of Johansen (2005), who considered the genus to have dispersed from north-eastern Sundaland to the current range. However, in our analyses the widespread clade is shown to have a wide ancestral distribution, whereas Johansen implied that this clade diversified in the Thai-Malay Peninsula. This is most likely because Johansen’s study did not include the Bornean and Indochinese species in this clade (O. jiewhoei and O. laotica, respectively).
The main differences between our study and Johansen’s (2005) are therefore likely to be in our wider taxonomic sampling, driven largely by species discovery and further collection in the field. To test the effect of species discovery in the DEC regions, we tried running the ancestral region reconstruction without recently (2020–2021) described species (Supplementary Figure 44). This had an effect on the reconstruction: the status of the stem and crown nodes of the Indochina + widespread clade changed, as did the basal nodes in the widespread clade. The potentially long-lasting connection between Borneo and the Thai-Malay Peninsula was not seen in these results. In families like Lowiaceae, where even major clades have no morphological synapomorphies, it is important to sample thoroughly even within morphologically and geographically similar groups when conducting biogeographic analyses.
The geological history of the distribution area of Lowiaceae is characterized by changes in the land masses of Sundaland (Thai-Malay Peninsula, Borneo, Sumatra, and Java) and has been affected by tectonic movement, sea-level changes (Hall, 2012) and climate (De Bruyn et al., 2014). The entire area was a continuous landmass with a humid and warm climate, until the separation of Indochina from Borneo starting c. 20–15 MYA. The current separation of Borneo from Mainland Asia is recent, only occurring c. 5 MYA. The shallow seas separating Borneo from the Thai-Malay Peninsular recede when sea levels decrease during glacial maxima, including during the last ice age. The long, continuous emergence of Borneo and Indochina likely explains the high biodiversity in these areas (De Bruyn et al., 2014). Lowiaceae are only found in these two areas and in Southern China and Peninsular Malaysia, all areas with a similar, continuous emergence; they notably absent from the Malesian islands other than Borneo. This distribution pattern is rare. Of the genera listed in Joyce et al. (2020), only 91 (1.77%) are present in Borneo and continental Asia but not on other islands. Several of those genera are also found outside of Malesia. In Zingiberales, the pattern is listed for three genera, but these do not hold to closer scrutiny, because these three genera also extend to other parts of Malay Archipelago.
A pattern of dispersal from Indochina and Borneo to other parts of Southeast Asia has been frequently observed in the region’s flora and fauna (De Bruyn et al., 2014). The entire distribution area of Lowiaceae could have been in a single landmass, Sundaland, for much of the evolutionary history of the family. Indochina and Borneo were directly connected until c. 20–15 MYA, and a continuous landmass existed between Borneo and the Thai-Malay Peninsula until c. 5 MYA (Hall, 2012). The dates could explain the relative geographic isolation of the Indochina clade, and the wide distribution of the widespread clade, although the reason for the limited distribution of the Borneo clade remains elusive from a geological perspective. It is somewhat unusual that no species in Lowiaceae is present in both Borneo and Continental Asia, given that the areas were connected during glacial maxima. In fact, all current species of Orchidantha have a very limited distribution, and it seems likely that dispersal events in Orchidantha are rare, perhaps leading to reproductive isolation of populations, and speciation. Long-distance dispersal events seem to be extremely rare in the genus; even the dispersal events suggested by our DEC + J analysis could be explained by dispersal of lineages along land corridors. Seed dispersal in Orchidantha has not been studied, but the seeds have no obvious structures that attract animals. Their fruits are partly embedded in the ground. Unlike some other Zingiberales with fruits in the ground, the fruits do not have a distinctive scent or fleshy parts. The seeds have an aril that forms multiple rigid coils which may act as springs, and help in short-distance dispersal of the seeds, but there are no known dispersal agents that could aid in long-distance dispersal. The seeds germinate soon after reaching the soil, Sometimes still while being trapped in the fruit, and like most Zingiberales, have rather limited longevity.
Few studies so far have focused on biogeography of forest plant genera within the Malesian and Indochinese floristic regions. De Bruyn et al. (2014) list six case studies [Aglaia Lour., Alocasia (Schott) G.Don, Begonia L., Cyrtandra J.R.Forst. and G.Forst. Rhododendron L., Rafflesiaceae], three of which were first published therein. Some of these studies have been superseded by more detailed analyses, and a few unrelated studies have been published since. Of these, Rafflesiaceae has similar dispersal ability to Lowiaceae, and it has since been studied in more detail. The species of Rafflesia R.Br. show similarly restricted distribution and strong geographic patterns to Orchidantha, perhaps also due to limited dispersal ability caused by ant dispersal (Pelser et al., 2019). Further studies also exist in Cyrtandra. The genus has evidently spread from Borneo to other areas, including independent range extensions to Thailand and Peninsular Malaysia, within the last c. 4–10 million years (Atkins et al., 2020). A similar pattern has been observed in Alocasia (Nauheimer et al., 2012) and Musa Colla (Janssens et al., 2016). Many genera in the Melastomataceae tribe Dissochaeteae seem to have had ancestral ranges from Borneo to mainland Asia, but the geographic patterns within these genera are less clear (Abdulrokhman Kartonegoro et al., 2021). Begonia (Thomas et al., 2012), Trigonostemon Blume and Dimorphocalyx Thwaites (Yu and van Welzen, 2020) have dispersed from mainland Asia into Malesia and then east. Rhododendron section Vireya has dispersed from the Sahul Shelf northwards, but the range extensions to Borneo and Peninsular Malaysia seem to be independent events, and the phylogeny is undated (Brown et al., 2006). Aglaia is likely a much older genus than Orchidantha, but it’s possible that some lineages in the genus moved from Borneo to Indochina c. 8–15 MYA (Muellner et al., 2008). All of these genera have a much wider insular distribution than Lowiaceae, and in dispersal ability, only Rafflesia is likely to be directly comparable to Lowiaceae.
Conclusion
Lowiaceae follows a general pattern that has been observed in phylogenetic studies in Malesia: biodiversity has spread from Borneo and Indochina to the rest of Southeast Asia. However, Lowiaceae is an unusual family in that the distribution stopped at the southern and central Thai-Malay Peninsula, with no known dispersal to the seasonal parts of Thai-Malay Peninsula, or to any other part of insular Malesia apart from Borneo. It is possible to explain the distribution of the genus entirely by land mass distribution in the past 15 million years, and it seems that the current range of the genus is a remnant of a once wider distribution initially covering north-eastern Sundaland and later a land bridge between Borneo and Thai-Malay Peninsula. All the extant species have a very small distribution area, and even the major clades are almost completely limited to single landmasses. Together, these patterns indicate that Lowiaceae have a very limited dispersal ability and partly explain the highly species-specific relationship with their pollinators.
Data Availability Statement
The datasets presented in this study can be found in online repositories. The names of the repository/repositories and accession number(s) can be found below: https://www.ncbi.nlm.nih.gov/, PRJNA668822. Target and bait sequences, assembled reads, alignments and tree files are available at https://doi.org/10.5061/dryad.h70rxwdkp.
Author Contributions
GK, JL-Š, and MN designed the study. GK secured the funding. AP, JL-Š, and MN conducted the field work and sample collection. JL-Š provided morphological data scoring. MN conducted the laboratory work. EG and MN conducted the phylogenetic analyses. OŠ conducted all genome size measurements and analyses. All authors were involved in writing, reading and approving the manuscript.
Funding
GK, MN, and JL-Š were supported by the National Parks Board. OŠ was financially supported by the Ministry of Culture of the Czech Republic (nos. 00023272, DKRVO 4.II.c). EG was supported by the United States National Science Foundation (DBI award no. 1711391).
Conflict of Interest
The authors declare that the research was conducted in the absence of any commercial or financial relationships that could be construed as a potential conflict of interest.
Publisher’s Note
All claims expressed in this article are solely those of the authors and do not necessarily represent those of their affiliated organizations, or those of the publisher, the editors and the reviewers. Any product that may be evaluated in this article, or claim that may be made by its manufacturer, is not guaranteed or endorsed by the publisher.
Acknowledgments
Wong Wei Hui, Koh Xin Yi, and Chong Shu Chen are acknowledged for their help during counting chromosomes of several species. We thank the following Botanic Gardens, institutions and individuals for plant exchanges and/or gifts of Lowiaceae material to Singapore Botanic Gardens without which this study would not have been possible: Tenom Agriculture Park (Sabah, Malaysia); Forest Research Institute Malaysia; South China Botanic Garden; Pha Tad Ke Botanic Garden and living collections of the Biotechnology and Ecology Institute, Ministry of Science and Technology (Lao P.D.R); Tan Jiew Hoe. We also thank local authorities for issuing permits, and all the staff from various institutions, national parks and forest departments who helped us during our field trips in Lao P.D.R (Pha Tad Ke Botanic Garden, Luang Prabang; Biotechnology and Ecology Institute, Ministry of Science and Technology, Vientiane), Vietnam [University of Science, Vietnam National University, fieldwork carried under the Sud Expert Plantes project (SEP project 350)], and Sarawak (Sarawak Forest Department and National Parks of Sarawak). We also thank Trần Hũˈu Ðăng, Aung Thame and Sarah Lim, the two former and the current managers of the research living collections at the Singapore Botanic Gardens, for their dedication in caring for the plants under their care. We thank Khoo-Woon Mui Hwang, the lab manager of the Molecular Biology Lab at the Singapore Botanic Gardens, for procuring the necessary materials and keeping the Lab in excellent order. We thank The Morton Arboretum and Case Western Reserve University for access to computational resources.
Supplementary Material
The Supplementary Material for this article can be found online at: https://www.frontiersin.org/articles/10.3389/fevo.2021.794977/full#supplementary-material
Supplementary Figure 1 | Sample of FCM histograms.
Supplementary Figure 2 | Chromosome counts of selected species.
Supplementary Figures 3–5 | Chronogram phylogenies.
Supplementary Figures 6–7 | Non-metric multidimensional scaling of non-genomic character states.
Supplementary Figures 8–27 | Ancestral state reconstruction of categorical morphological character states.
Supplementary Figures 28–42 | Ancestral state reconstruction of quantitative morphological character states.
Supplementary Figure 43 | Biogeographical analysis using DEC+J in BioGeoBEARS.
Supplementary Figure 44 | Effect of species discovery on the ancestral range reconstruction.
Supplementary Table 1 | Voucher information of samples used in this study.
Supplementary Table 2 | Morphological matrix.
Supplementary Table 3 | Chromosome counts and genome sizes.
References
Atkins, H. J., Bramley, G. L. C., Johnson, M. A., Kartonegoro, A., Nishii, K., Kokubugata, G., et al. (2020). A molecular phylogeny of Southeast Asian Cyrtandra (Gesneriaceae) supports an emerging paradigm for Malesian plant biogeography. Front. Biogeogr. 12:e44814. doi: 10.21425/F5FBG44184
Barrett, C. F., Specht, C. D., Leebens-Mack, J., Stevenson, D. W., Zomlefer, W. B., and Davis, J. I. (2014). Resolving ancient radiations: can complete plastid gene sets elucidate deep relationships among the tropical gingers (Zingiberales)? Ann. Bot. 113, 119–133. doi: 10.1093/aob/mct264
Beath, D. N. (1996). Pollination of Amorphophallus johnsonii (Araceae) by carrion beetles (Phaeochrous amplus) in a Ghanaian rain forest. J. Trop. Ecol. 12, 409–418.
Beath, D. N. (1999). Dynastine scarab beetle pollination in Dieffenbachia longispatha (Araceae) on Barro Colorado Island (Panama) compared with La Selva biological station (Costa Rica). Aroideana 22, 63–71.
Beaulieu, J. M., Leitch, I. J., and Knight, C. A. (2007). Genome size evolution in relation to leaf strategy and metabolic rates revisited. Ann. Bot. 99, 495–505. doi: 10.1093/aob/mcl271
Bharathan, G., Lambert, G., and Galbraith, D. W. (1994). Nuclear DNA content of Monocotyledons and related taxa. Amer. J. Bot. 81, 381–386. doi: 10.1002/j.1537-2197.1994.tb15457.x
Bolger, A. M., Lohse, M., and Usadel, B. (2014). Trimmomatic: a flexible trimmer for Illumina sequence data. Bioinformatics 30, 2114–2120. doi: 10.1093/bioinformatics/btu170
Brown, G. K., Nelson, G., and Ladiges, P. Y. (2006). Historical biogeography of Rhododendron section Vireya and the Malesian Archipelago. J. Biogeogr. 33, 1929–1944. doi: 10.1111/j.1365-2699.2006.01548.x
Brown, N. E. (1886). Orchidantha borneensis N.E.Brown [A new genus of Scitamineae]. Gard. Chron. 26:519.
Capella-Gutiérrez, S., Silla-Martínez, J. M., and Gabaldón, T. (2009). trimAl: a tool for automated alignment trimming in large-scale phylogenetic analyses. Bioinformatics 25, 1972–1973. doi: 10.1093/bioinformatics/btp348
Carlsen, M. M., Fér, T., Schmickl, R., Leong-Škorničková, J., Newman, M., and Kress, J. W. (2018). Resolving the rapid plant radiation of early diverging lineages in the tropical Zingiberales: pushing the limits of genomic data. Mol. Phylogenet. Evol. 128, 55–68. doi: 10.1016/j.ympev.2018.07.020
De Bruyn, M., Stelbrink, B., Morley, R. J., Hall, R., Carvalho, G. R., Cannon, C. H., et al. (2014). Borneo and Indochina are major evolutionary hotspots for Southeast Asian biodiversity. Syst. Biol. 63, 879–901. doi: 10.1093/sysbio/syu047
Doležel, J., Greilhuber, J., Lucretti, S., Meister, A., Lysák, M. A., Nardi, L., et al. (1998). Plant genome size estimation by flow cytometry: inter-laboratory comparison. Ann. Bot. 82, 17–26. doi: 10.1006/anbo.1998.0730
Doyle, J. J., and Doyle, J. L. (1987). A rapid DNA isolation procedure for small quantities of fresh leaf tissue. Phytochem. Bull. 19, 11–15.
Faegri, K., and van der Pijl, L. (1971). The Principles of Pollination Ecology. New York, NY: Pergamon.
Faircloth, B. C., McCormack, J. E., Crawford, N. G., Harvey, M. G., Brumfield, R. T., and Glenn, T. C. (2012). Ultraconserved elements anchor thousands of genetic markers spanning multiple evolutionary timescales. Syst. Biol. 61, 717–726. doi: 10.1093/sysbio/sys004
Gibernau, M., Macquart, D., and Przetak, G. (2004). Pollination in the genus Arum: a review. Aroideana 27, 148–166.
Givnish, T. J., Pires, J. C., Graham, S. W., McPherson, M. A., Prince, L. M., Patterson, T. B., et al. (2006). Phylogenetic relationships of monocots based on the highly informative plastid gene ndhF: evidence for widespread concerted convergence. Aliso 22, 28–51. doi: 10.5642/aliso.20062201.04
Goodrich, K. R., and Raguso, R. A. (2009). The olfactory component of floral display in Asimina and Deeringothamnus (Annonaceae). New Phytol. 183, 457–469. doi: 10.1111/j.1469-8137.2009.02868.x
Greilhuber, J., Temsch, E. M., and Loureiro, J. C. M. (2007). “Nuclear DNA content measurement,” in Flow Cytometry with Plant Cells, eds J. Doležel, J. Greilhuber, and J. Suda (Weinheim: Wiley), 67–101.
Grimm, R. (2009). Peltonotus nasutus Arrow, 1910 and species of Phaeochrous as pollinators of Amorphophallus paeoniifolius (Araceae) in Thailand (Coleoptera: Scarabaeidae). Entomol. Z. Insekten Borse 119, 167–168.
Hall, I. (2012). “Sundaland and Wallacea: geology, plate tectonics and palaeogeography,” in Biotic Evolution and Environmental Change in Southeast Asia, eds D. Gower, K. Johnson, J. Richardson, B. Rosen, L. Rüber, S. Williams, et al. (Cambridge: Cambridge University Press).
Harris, L. W., and Davies, T. J. (2016). A complete fossil-calibrated phylogeny of seed plant families as a tool for comparative analyses: testing the ‘time for speciation’ hypothesis. PLoS One 12:e0172816. doi: 10.1371/journal.pone.0162907
Heibl, C. (2008). PHYLOCH: R Language Tree Plotting Tools and Interfaces to Diverse Phylogenetic Software Packages. Available online at www.christophheibl.de/Rpackages.html (accessed June 3, 2021).
Janssens, S. B., Vandelook, F., De Langhe, E., Verstraete, B., Smets, E., Vandenhouwe, I., et al. (2016). Evolutionary dynamics and biogeography of Musaceae reveal a correlation between the diversification of the banana family and the geological and climatic history of Southeast Asia. New Phytol. 210, 1453–1465. doi: 10.1111/nph.13856
Jenjittikul, T., and Larsen, K. (2003). Orchidantha foetida (Lowiaceae) a new species from Thailand. Nord. J. Bot. 22, 405–408. doi: 10.1111/j.1756-1051.2002.tb01391.x
Johansen, L. B. (2005). Phylogeny of Orchidantha (Lowiaceae) and the Zingiberales based on six DNA Regions. Syst. Bot. 30, 106–117. doi: 10.1600/0363644053661931
Johnson, M. G., Gardner, E. M., Liu, Y., Medina, R., Goffinet, B., Shaw, A. J., et al. (2016). HybPiper: extracting coding sequence and introns for phylogenetics from high-throughput sequencing reads using target enrichment. Appl. Plant Sci. 4:as.1600016. doi: 10.3732/apps.1600016
Joyce, E. M., Thiele, K. R., Slik, F. J. W., and Crayn, D. M. (2020). Checklist of the vascular flora of the Sunda-Sahul convergence zone. Biodivers. Data J. 8:e51094. doi: 10.3897/BDJ.8.e51094
Kartonegoro, A., Mota de Oliveira, S., and van Welzen, P. C. (2021). Historical biogeography of the Southeast Asian and Malesian tribe Dissochaeteae (Melastomataceae). J. Syst. Evol. doi: 10.1111/jse.12752
Katoh, K., and Standley, D. M. (2013). MAFFT multiple sequence alignment software version 7: improvements in performance and usability. Mol. Biol. Evol. 30, 772–780. doi: 10.1093/molbev/mst010
Kent, W. J. (2002). BLAT – the BLAST-like alignment tool. Genome Res. 12, 656–664. doi: 10.1101/gr.229202.
Kirchoff, B. K., Liu, H., and Liao, J.-P. (2020). Inflorescence and flower development in Orchidantha chinensis T.L. Wu (Lowiaceae; Zingiberales): similarities to inflorescence structure in the Strelitziaceae. Int. J. Plant Sci. 181, 716–731. doi: 10.1086/709296
Knight, C. A., Molinari, N. A., and Petrov, D. A. (2005). The large genome constraint hypothesis: evolution, ecology and phenotype. Ann. Bot. 95, 177–190. doi: 10.1093/aob/mci011
Kress, W. J. (1990). The phylogeny and classification of the Zingiberales. Ann. Missouri Bot. Gard. 77, 698–721. doi: 10.2307/2399669
Kress, W. J., and Specht, C. D. (2006). The evolutionary and biogeographic origin and diversification of the tropical monocot order Zingiberales. Aliso 22, 621–632. doi: 10.5642/aliso.20062201.49
Kress, W. J., Prince, L. M., Hahn, W. J., and Zimmer, E. A. (2001). Unraveling the evolutionary radiation of the families of the Zingiberales using morphological and molecular evidence. Syst. Biol. 50, 926–944. doi: 10.1080/106351501753462885
Larsen, K. (1966). Chromosome cytology and relationship of the Lowiaceae. Nat. Hist. Bull. Siam. Soc. 21, 21–24.
Larsen, K. (1993). A new species of Orchidantha (Lowiaceae) from Borneo. Nord. J. Bot. 13, 285–288. doi: 10.1111/j.1756-1051.1993.tb00050.x
Larsen, K. (1996). “Lowiaceae,” in Flora Malesiana I, Vol. 12, eds C. Kalkman, P. F. Stevens, D. W. Kirkup, W. J. J. O. de Wilde, and H. P. Nooteboom (Leiden: Flora Malesiana Foundation), 763–774.
Leebens-Mack, J. H., Barker, M. S., Carpenter, E. J., Deyholos, M. K., Gitzendanner, M. A., Graham, S. W., et al. (2019). One thousand plant transcriptomes and the phylogenomics of green plants. Nature 574, 679–685. doi: 10.1038/s41586-019-1693-2
Leitch, I. J., Johnston, E., Pellicer, J., Hidalgo, O., and Bennett, M. D. (2019). Plant DNA C-Values Database. Available online at: https://cvalues.science.kew.org/ (accessed April, 2019).
Leong-Škorničková, J. (2014). Orchidantha lengguanii (Lowiaceae), a new species from Peninsular Malaysia, and typification of O. maxillarioides. Gard. Bull. Singapore 66, 15–25.
Leong-Škorničková, J., Lamb, A., Linton, J., and Gokusing, L. (2021). Six new Orchidantha species (Lowiaceae) from Borneo. Gard. Bull. Singapore 73, 179–202. doi: 10.26492/gbs73(1).2021-08
Leong-Škorničková, J., Nguyễn, Q. B., and Šída, O. (2014). Orchidantha virosa Škorničk. & Q.B.Nguyễn, sp. nov. (Lowiaceae), a new species endemic to northern Vietnam. Adansonia 36, 237–243.
Liang, H., Zhang, Y., Deng, J., Gao, G., Ding, C., Zhang, L., et al. (2020). The complete chloroplast genome sequences of 14 Curcuma species: insights into genome evolution and phylogenetic relationships within Zingiberales. Front. genet. 11:802. doi: 10.3389/fgene.2020.00802
Mahanty, H. K. (1970). A cytological study of the Zingiberales with special reference to their taxonomy. Cytologia 35, 13–49. doi: 10.1508/cytologia.35.13
Mandel, J. R., Dikow, R. B., Funk, V. A., Masalia, R. R., Staton, S. E., Kozik, A., et al. (2014). A target enrichment method for gathering phylogenetic information from hundreds of loci: an example from the compositae. Appl. Plant Sci. 2:1300085. doi: 10.3732/apps.1300085
Matzke, N. J. (2013). Probabilistic historical biogeography: new models for founder-event speciation, imperfect detection, and fossils allow improved accuracy and model-testing. Front. Biogeogr. 5:242. doi: 10.21425/F5FBG19694
Morgan, H. D., and Westoby, M. (2005). The relationship between nuclear DNA content and leaf strategy in seed plants. Ann. Bot. 96, 1321–1330. doi: 10.1093/aob/mci284
Muellner, A. N., Pannell, C. M., Coleman, A., and Chase, M. W. (2008). The origin and evolution of Indomalesian, Australasian and Pacific island biotas: insights from Aglaieae (Meliaceae, Sapindales). J. Biogeogr. 35, 1769–1789. doi: 10.1111/j.1365-2699.2008.01935.x
Nagamasu, H., and Sakai, S. (1999). Orchidantha inouei (Lowiaceae), a new species from Borneo. Nord. J. Bot. 19, 149–152. doi: 10.1111/j.1756-1051.1999.tb00658.x
Nauheimer, L., Boyce, P. C., and Renner, S. S. (2012). Giant taro and its relatives: a phylogeny of the large genus Alocasia (Araceae) sheds light on Miocene floristic exchange in the Malesian region. Mol. Phylogenet. Evol. 63, 43–51. doi: 10.1016/j.ympev.2011.12.01
Niissalo, M. A., Leong-Škorničková, J., Šída, O., and Khew, G. S. (2020). Population genomics reveal apomixis in a novel system: uniclonal female populations dominate the tropical forest herb family, Hanguanaceae (Commelinales). AoB PLANTS 12:laa053. doi: 10.1093/aobpla/plaa053
Oksanen, J., Guillaume Blanchet, F., Friendly, M., Kindt, R., Legendre, P., McGlinn, D., et al. (2020). vegan: Community Ecology Package. R Package Version 2.5-7. Available onlline at: https://CRAN.R-project.org/package=vegan (accessed Nov 28, 2020).
Otto, F., Crissman, H. A., and Darzynkiewicz, Z. (1990). DAPI staining of fixed cells for high-resolution flow cytometry of nuclear DNA. Methods Cell Biol. 33, 105–110. doi: 10.1016/s0091-679x(08)60516-6
Paradis, E. (2013). Molecular dating of phylogenies by likelihood methods: a comparison of models and a new information criterion. Mol. Phylogenet. Evol. 67, 436–444. doi: 10.1016/j.ympev.2013.02.008
Paradis, E., and Schliep, K. (2019). ape 5.0: an environment for modern phylogenetics and evolutionary analyses in R. Bioinformatics 35, 526–528. doi: 10.1093/bioinformatics/bty633
Pedersen, L. B. (2001). Four new species of Orchidantha (Lowiaceae) from Sabah. Nord. J. Bot. 21, 121–128. doi: 10.1111/j.1756-1051.2001.tb01346.x
Pedersen, L. B. (2003). Phylogeny and Pollination Biology of Members of Zingiberales, with Special Emphasis on Lowiaceae. Unpublished Ph.D. thesis. Copenhagen: University of Copenhagen.
Pedersen, L. B., and Johansen, B. (2004). Anatomy of the unusual stigma in Orchidantha (Lowiaceae) Am. J. Bot. 91, 299–305. doi: 10.3732/ajb.91.3.299
Pelser, P. B., Nickrent, D. L., van Eec, B. W., and Barcelona, J. F. (2019). A phylogenetic and biogeographic study of Rafflesia (Rafflesiaceae) in the Philippines: limited dispersal and high island endemism. Mol. Phylogenet. Evol. 139:106555. doi: 10.1016/j.ympev.2019.106555
Poulsen, A. D., and Leong-Škorničková, J. (2017). Two new Orchidantha species (Lowiaceae) from Borneo. Blumea 62, 157–162. doi: 10.3767/blumea.2017.62.02.08
R Development Core Team (2019). R: A Language and Environment for Statistical Computing. Vienna: R Foundation for Statistical Computing.
Ree, R. H., and Sanmartín, I. (2018). Conceptual and statistical problems with the DEC+J model of founder-event speciation and its comparison with DEC via model selection. J. Biogeogr. 45, 741–749. doi: 10.1111/jbi.13173
Revell, L. J. (2012). phytools: an R package for phylogenetic comparative biology (and other things). Methods Ecol. Evol. 3, 217–223. doi: 10.1111/j.2041-210X.2011.00169.x
Sakai, S., and Inoue, T. (1999). A new pollination system: dung-beetle pollination discovered in Orchidantha inouei (Lowiaceae, Zingiberales) in Sarawak, Malaysia. Amer. J. Bot. 86, 56–61.
Sanderson, M. J. (2002). Estimating absolute rates of molecular evolution and divergence times: a penalized likelihood approach. Mol. Biol. Evol. 19, 101–109. doi: 10.1093/oxfordjournals.molbev.a003974
Sass, C., Iles, W. J. D., Barrett, C. F., Smith, S. Y., and Specht, C. D. (2016). Revisiting the Zingiberales: using multiplexed exon capture to resolve ancient and recent phylogenetic splits in a charismatic plant lineage. PeerJ 4:e1584. doi: 10.7717/peerj.1584
Sayyari, E., and Mirarab, S. (2018). Testing for polytomies in phylogenetic species trees using quartet frequencies. Genes 9:132. doi: 10.3390/genes9030132
Schliep, K. P. (2011). phangorn: phylogenetic analysis in R. Bioinformatics 27, 592–593. doi: 10.1093/bioinformatics/btq706
Schmickl, R., Liston, A., Zeisek, V., Oberlander, K., Weitemier, K., Straub, S. C., et al. (2016). Phylogenetic marker development for target enrichment from transcriptome and genome skim data: the pipeline and its application in southern African Oxalis (Oxalidaceae). Mol. Ecol. Resour. 16, 1124–1135. doi: 10.1111/1755-0998.12487
Setiawan, A. G., Teo, C. H., Kikuchi, S., Sassa, H., and Koba, T. (2018). An improved method for inducing prometaphase chromosomes in plants. Mol. Cytogenet. 11:32. doi: 10.1186/s13039-018-0380-6
Šmarda, P., Bureš, P., Horová, L., Leitch, I. J., Mucina, L., Pacini, L., et al. (2014). Ecological and evolutionary significance of genomic GC content diversity in monocots. Proc. Natl. Acad. Sci. U.S.A. 111, E4096–E4102. doi: 10.1073/pnas.1321152111
Smith, J. F., Kress, W. J., and Zimmer, E. A. (1993). Phylogenetic analysis of the Zingiberales based on rbcL sequences. Ann. Missouri Bot. Gard. 80, 620–630. doi: 10.2307/2399850
Smith, S. A., and O’Meara, B. C. (2012). Divergence time estimation using penalized likelihood for large phylogenies. Bioinformatics 28, 2689–2690. doi: 10.1093/bioinformatics/bts492
Song, J. J., Liao, J. P., Tang, Y. J., and Chen, Z. Y. (2004). Chromosome numbers in Orchidantha (Lowiaceae) and their biogeographic and systematic implications. Ann. Bot. Fenn. 41, 429–433.
Song, J. J., Tang, Y. J., Liao, J. P., Huang, X. X., and Chen, Z. Y. (2003). Chromosome numbers of Orchidantha (Lowiaceae). Acta Bot. Yunnan. 25, 609–612.
Stamatakis, A. (2014). RAxML version 8: a tool for phylogenetic analysis and post-analysis of large phylogenies. Bioinformatics 30, 1312–1313. doi: 10.1093/bioinformatics/btu033
Syauqina, M. Y., Meekiong, K., and Aimi-Syazana, S. (2019). Orchidantha sarawakensis sp. nov. (Zingiberales: Lowiaceae), a new species endemic to East Malaysia, Borneo. J. Trop. For. Sci. 31, 371–376. doi: 10.26525/jtfs2019.31.3.371
Syauqina, M. Y., Meekiong, K., Tawan, C. S., and Syazana, S. A. (2016). Orchidantha ranchanensis, a new species of Lowiaceae from Sarawak, Malaysia. Folia Malaysiana 17, 35–42.
Takhtajan, A. (1986). Floristic Regions of the World (Translation from Russian), trans. T. J. Crovello, A. Cronquist. Berkeley: University of California Press.
Thomas, D. C., Hughes, M., Phutthai, T., Ardi, W. H., Rajbhandary, S., Rubite, R., et al. (2012). West to east dispersal and subsequent rapid diversification of the mega-diverse genus Begonia (Begoniaceae) in the Malesian archipelago. J. Biogeogr. 39, 98–113. doi: 10.1111/j.1365-2699.2011.02596.x
Trần, H. Ð, and Leong-Škorničková, J. (2010). Orchidantha stercorea sp. nov. (Lowiaceae) from Vietnam. Nord. J. Bot. 28, 299–303. doi: 10.1111/j.1756-1051.2009.00704.x
Trần, H. Ð, Lúu, H. T., and Leong-Škorničková, J. (2020). Orchidantha anthracina (Lowiaceae), a new species from Vietnam. Blumea 65, 90–93. doi: 10.3767/blumea.2020.65.01.12
Vislobokov, N. A., Nuraliev, M. S., and Galinskaya, T. V. (2017). Pollination ecology of Lowiaceae (Zingiberales): nocturnal carrion-beetle pollination of Orchidantha virosa. Int. J. Plant Sci. 178, 302–312. doi: 10.1086/690910
Weitemier, K., Straub, S. C. K., Cronn, R. C., Fishbein, M., Schmickl, R., McDonnell, A., et al. (2014). Hyb-Seq: combining target enrichment and genome skimming for plant phylogenomics. Appl. Pl. Sci. 2:1400042. doi: 10.3732/apps.1400042
Welk, E. (2015). “Phytogeography of the Nepalese flora and its floristic links to neighbouring regions,” in Nepal: An Introduction to the Natural History, Ecology and Human Environment of the Himalayas. A Companion to the Flora of Nepal, eds G. Miehe, C. Pendry, and R. Chaudhary (Edinburgh: Royal Botanic Garden Edinburgh).
Yu, R.-Y., and van Welzen, P. C. (2020). Historical biogeography of Trigonostemon and Dimorphocalyx (Euphorbiaceae). Bot. J. Linn. Soc. 192, 333–349. doi: 10.1093/botlinnean/boz075
Zhang, C., Rabiee, M., Sayyari, E., and Mirarab, S. (2018). ASTRAL-III: polynomial time species tree reconstruction from partially resolved gene trees. BMC Bioinformatics 19:153. doi: 10.1186/s12859-018-2129-y
Zou, P., Cai, X. A., Xu, K., Cui, C. J., Ye, Y. S., and Liao, J. P. (2019). Orchidantha crassinervia sp. nov. (Lowiaceae) from Guangxi. China. Nord. J. Bot. 37, 1–5.
Keywords: biogeography, genome size, HybSeq, Orchidantha, polyploidy, Southeast Asia, Zingiberales, Lowiaceae
Citation: Niissalo MA, Gardner EM, Khew GS, Šída O, Poulsen AD and Leong-Škorničková (2022) Whence Came These Plants Most Foul? Phylogenomics and Biogeography of Lowiaceae (Zingiberales). Front. Ecol. Evol. 9:794977. doi: 10.3389/fevo.2021.794977
Received: 14 October 2021; Accepted: 29 November 2021;
Published: 05 January 2022.
Edited by:
Ferhat Celep, Kırıkkale University, TurkeyReviewed by:
Jeffrey P. Rose, University of Nebraska at Kearney, United StatesChun-Lei Xiang, Kunming Institute of Botany, Chinese Academy of Sciences (CAS), China
Copyright © 2022 Niissalo, Gardner, Khew, Šída, Poulsen and Leong-Škorničková. This is an open-access article distributed under the terms of the Creative Commons Attribution License (CC BY). The use, distribution or reproduction in other forums is permitted, provided the original author(s) and the copyright owner(s) are credited and that the original publication in this journal is cited, in accordance with accepted academic practice. No use, distribution or reproduction is permitted which does not comply with these terms.
*Correspondence: Matti A. Niissalo, Matti_Asser_Niissalo@nparks.gov.sg; Jana Leong-Škorničková, Jana_Skornickova@nparks.gov.sg
†These authors have contributed equally to this work and share first authorship